Jesse H. Ausubel
50th Anniversary Symposium of the Geology Foundation
Jackson School of Geosciences, U. of Texas
Austin, Texas
25 April 2003
Introduction
About 750,000 years ago some of our ancestors made a wood fire in a cave in the south of France near Marseilles. From such early fires until about the year 1800 energy supply changed little. The system relied on carbon, like a mesquite grill.
The most important and surprising fact to emerge from energy studies during the past two decades is that, for the last 200 years, the world has progressively pursued a path of decarbonization, a decreasing relative reliance on carbon [Figure 1]. Think of decarbonization as the course over time in the ratio of tons of carbon in the energy supply to the total energy supply, for example, tons of carbon per tons of oil equivalent encompassing all energy supplies.
Alternately, think hydrocarbons. Both hydrogen and carbon burn to release heat, so we can consider decarbonization as the ratio of hydrogen and carbon in our bowl of energy chili. When the energy system relied on hay and wood, it relied most heavily on carbon. Wood is made of much cellulose and some lignin. Heated cellulose leaves charcoal, almost pure carbon. Lignin is a hydrocarbon with a complex benzenic structure. Wood effectively burns about ten carbon for each hydrogen atom. Coal approaches parity with one or two C’s per H, depending on the variety [Figure 2]. Oils are lighter yet, with, for example, with two H’s per C, in kerosene or jet fuel. A molecule of methane, the typical natural gas, is a carbon-trim CH4.
Thus, the inverse of decarbonization is the ascendancy of hydrogen [Figure 3]. Think of hydrogen and carbon competing for market niche as did horses and automobiles or audio cassettes and compact discs, except the H/C competition extends over 300 years. In 1800 carbon had 90% of the market. In 1935 the elements tied. With business as usual, hydrogen will garner 90% of the market around 2100.
Because carbon becomes soot or the feared greenhouse gas CO2, and hydrogen becomes only water when combusted, carbon appears a bad element, the black hat, and hydrogen a good one, the white hat. So, decarbonization is not only a fact but a happy fact.
Let me explain the course of decarbonization. Neither Thomas Jefferson nor Queen Victoria decreed it. Why does it happen? The driving force in evolution of the energy system is the increasing spatial density of energy consumption at the level of the end user.
By 1800 or so, in England and other early loci of industry, high population density and the slow but steady increase in energy use per capita increased the density of energy consumption. The British experience demonstrates that, when energy consumption per unit of area rises, the energy sources with higher economies of scale gain an advantage.
Wood and hay, the prevalent energy sources at the start of the 19th century, are bulky and awkward to transport and store. Consider the outcome if every high-rise resident needed to keep both a cord of wood on her floor for heat and a pile of hay in the garage for the SUV. Think of retailing these goods in the costly real estate of Dallas or New York. Sales of fuel wood in cities now are, of course, limited to decorative logs providing emotional warmth. Biomass gradually lost the competition with coal to fuel London and other multiplying and concentrating populations, even when wood was abundant.
Coal had a long run at the top of the energy heap. It ruled notwithstanding its devastating effects on miners’ lungs and lives, the urban air, and the land from which it came; but about 1900, the advantages of an energy system of fluids rather than solids began to become evident. On the privacy of its rails, a locomotive could pull a coal car of equal size to fuel it. Coal-powered automobiles, however, never had much appeal. The weight and volume of the fuel were hard problems, especially for a highly distributed transport system. Oil had a higher energy density than coal—and the advantage of flowing through pipelines and into tanks. Systems of tubes and cans can deliver carefully regulated quantities of fuel from the scale of the engine of a motor car to that of the Alaska pipeline. It is easy to understand why oil defeated coal by 1950 as the world’s leading energy source.
Yet, despite many improvements from wellhead to gasoline pump, distribution of oil is still clumsy. Fundamentally, oil is stored in a system of metal cans of all sizes. One famous can was the Exxon Valdez. Transfer between cans is imperfect, which brings out a fundamental point. The strongly preferred configuration for very dense spatial consumption of energy is a grid that can be fed and bled continuously at variable rates. There are two successful grids, gas and electricity.
Natural gas is distributed through an inconspicuous, pervasive, and efficient system of pipes. Its capillaries reach right to the kitchen. It provides an excellent hierarchy of storage, remaining safe in geological formations until shortly before use. Natural gas can be easily and highly purified, permitting complete combustion.
Electricity, which must be made from primary energy sources such as coal and gas, is both a substitute for these (as in space heating) and a unique wayto power devices that exist only because electricity became widely available. Electricity is an even cleaner energy carrier than natural gas and can be switched on and off with little effort and great effect. Electricity, however, continues to suffer a disadvantage: it cannot be stored efficiently, as today’s meager batteries show. Electrical losses also occurin transmission; with the present infrastructure, a distance of 100 km is normal for transmission, and about 1,000 km is the economic limit. Moreover, because of its limited storage, electricity is not good for dispersed uses, such as cars.
Nevertheless, the share of primary energy used to make electricity has grown steadily in all countries over the past 75 years and now approaches 40%. The Internet economy demands further electrification, with perfect reliability. Thus, the core energy game for the next 30 to 50 years is to expand and flawlessly operate the gas–electric system.
In contrast to what many believe, the stable dynamics of the energy system permit reliable forecasts. Decarbonization essentially defines the future of energy supply.
Globally we are destined to use about 50-80 billion tons more coal. This is about one-third what humans have mined in all our earlier history, and about 30 years at present levels of production, so all the participants in the coal industry have a generation or so in which to remodel themselves. We should squeeze the maximum electricity from the black rocks with the minimum fallout of nasties, but coal is not our primary concern because its use will fade anyway. In fact, coal companies would better concentrate on extracting methane from coal seams and sink CO2 there, staying in business without coal extraction. Using CO2 to displace methane (CH4)adsorbed in coal beds provides a two for one bargain. Tunneling, as we shall see, matters immensely for future human well being, so the coal industry also has a valuable skill to sell.
If it is dusk for coal, it is mid-afternoon for oil, which already has lost in energy markets other than transport. Globally, drivers and others will consume close to 300 billion tons more oil, before the fleet runs entirely on H2 separated from methane or water. This amount is almost double the petroleum that has so far been extracted, and about 50 years at present production, so oil companies can choose to play business as usual for a while. But the entry under the car’s hood of fuel cells or other motors fueled by H2 dooms oil, over the decades required for the turnover of the fleet, and makes a huge niche for the easy ways to make the needed hydrogen fuel.
For gas, it is midmorning, and the next decades will bring enormous growth, matching rising estimates of the gas resource base, which have more than doubled over the past 20 years. Preaching the advent of the Methane Age 20 years ago I felt myself a daring prophet but now this prophecy is like invoking the sunrise. Between its uses to fuel turbines to make electric power and for fuel cells for transport, gas will dominate the primary energy picture for the next five or six decades. I expect methane to provide perhaps 70% of primary energy soon after the year 2030 and to reach a peak absolute use in 2060 of about 30 x 1012 m3, ten times present annual use.
Through fuel cells we will adopt gas in transport as well as for electric power. Fuel cells, essentially continuous batteries, can be fed by hydrogen extracted from methane. In replacing the internal combustion engine, they will multiply automotive efficiencies and slash pollutants. Wood and coal fogged and blackened cities, and oil gave us brown clouds of smog; methane can complete the clearing of the skies of Houston and other cities in the world, soon to come, of one billion motor vehicles. Governments will need to make it easier to build and access gas pipelines. Attention must also be given to the safety and environmental aspects of gas use because pipelines and tanks can explode tragically. Refiners need to shift their focus to transforming methane into hydrogen and CO2.
Very Large ZEPPs
Now let me introduce the first of two Texas-size ideas. My first is zero emission electric power plants or ZEPPs, very large ZEPPs. The emission of concern is, of course, carbon, feared because of climate change. Although simply substituting methane for coal or oil reduces CO2 emissions by a third to a half, the peak use would correspond to 2 to 3 times today’s carbon emission to dispose annually. Even in 2020, we could already need to dispose carbon from natural gas alone equal to half today’s emission from all fuel and later methane would cause about 75% of total CO2 emissions. So, prevention of climate change must focus on methane. Can we find technology consistent with the evolution of the energy system to dispose economically and conveniently the carbon from making kilowatts? The practical means to dispose the carbon from generating electricity consistent with the future context is the very large ZEPP. Let me try to leave ZEPPs indelibly in your minds.
The basic idea of the ZEPP is a gas power plant operating at very high temperatures and pressures, so we can bleed off the CO2 as a liquid and sequester it underground in porous formations like those that harbor oil.
A criterion for ZEPPs is working on a Texas scale. One reason is the information economy. Even with efficiency increasing, the information economy demands huge amounts of electricity. Observe the recent rapid growth of demand in a college dormitory. Chips could well go into 1000 objects per capita, or 10 trillion objects, as China and India log into the game.
Big total energy use means big individual ZEPPs because the size of generating plants grows even faster than use, though in spurts. Plants grow because large is cheap, if technology can cope. Although the last wave of power station construction reached about 1.5 gigawatts (GW), growth of electricity use for the next 50 years can reasonably raise plant size to about 5 GW. For reference, my city, New York, now draws above 12 GW on a peak summer day.
Bigness is a plus for controlling emission. Although one big plant emits no more than many small plants, emission from one is easier to collect. Society cannot close the carbon cycle if we need to collect emissions from millions of microturbines.
Big ZEPPs means transmitting immense mechanical power from larger and larger generators through a large steel axle as fast as 3,000 revolutions per minute (RPM). The way around the limits of mechanical power transmission may be shrinking the machinery. Begin with a very high pressure CO2 gas turbine where fuel burns with oxygen. Needed pressure ranges from 40 to 1000 atmospheres, where CO2 would be recirculated as a liquid. The liquid combustion products would be bled out.
Fortunately for transmitting mechanical power, the high pressures shrink the machinery in a revolutionary way and so permits the turbine to rotate very fast. The generator could then also turn very fast, operating at high frequency, with appropriate power electronics to slow the generated electricity to 60 cycles.
Our envisioned hot temperature of 1500 degrees C will probably require using new ceramics now being engineered for aviation. Problems of stress corrosion and cracking will arise at the high temperatures and pressures and need to be solved. Power electronics to slow the cycles of the alternating current also raises big questions. What we envision is beyond the state of the art, but power electronics is still young, meaning expensive and unreliable, and we are thinking of the year 2020 and beyond.
The requisite oxygen for a 5 GW ZEPP also exceeds present capacity but could be made by cryoseparation. Moreover, the cryogenic plant may introduce a further benefit. Superconductors fit well with a cryogenic plant nearby. Superconducting generators are a sweet idea. Already today companies are selling small motors wound with high temperature superconducting wire that halve the size and weight of a conventional motor built with copper coils and also halve the electrical losses. Colleagues at Tokyo Electric Power calculate the overall ZEPP plant efficiency could be 70%, well above the 50-55% peak performance of today.
With a ZEPP fueled by natural gas transmitting immense power at 60 cycles, the next step is sequestering the waste carbon. At the high pressure, the waste carbon is, of course, already liquid carbon dioxide and thus easily-handled. Opportunity for storing CO2 will join access to customers and fuel in determining plant locations. Because most natural gas travels far through a few large pipelines, these pipelines are the logical sites for ZEPPs. The best way to sequester the emissions is in caverns underground, where coal, oil, and gas came from. On a small scale, CO2 already profitably helps tertiary recovery of oil. The challenge is large scale. The present annual volume of CO2 from all sources is about 15 km3. Of course natural geological traps only occasionally contain hydrocarbons, so one can extend storage to the traps that lack oil and gas that prospectors routinely find. Aquifers in silicate beds could be used to move the waste CO2 to the silicates where “weathering” would make carbonates and silica, an offset good for millions of years.
In short, the ZEPP vision is a supercompact, superpowerful, superfast turbine: 1-2 m diameter, potentially 10 GW or double the expected maximum demand, 30,000 RPMs, putting out electricity at 60 cycles plus CO2 that can be sequestered. ZEPPs the size of a locomotive or even an automobile, attached to gas pipelines, might replace the fleet of carbon emitting antiques now cluttering our landscape.
I propose starting introduction of ZEPPs in 2020, leading to a fleet of 500 5 GW ZEPPs by 2050. This does not seem an impossible feat for a world that built today’s worldwide fleet of some 430 nuclear power plants in about 30 years. ZEPPs, together with another generation of nuclear power plants in various configurations, can stop CO2 increase in the atmosphere near 2050 AD in the range 450-500 ppm, about one-quarter more than today, without sacrificing energy consumption.
ZEPPs merit tens of billions in R&D, because the plants will form a profitable industry worth much more to those who can capture the expertise to design, build, and operate them. They offer the best chance for safe use of the immense wealth of hydrocarbons in America and its offshore exclusive economic zones. Research on ZEPPs could occupy legions of academic researchers, and restore an authentic mission to the Department of Energy’s National Laboratories, working on development in conjunction with private companies. ZEPPs need champions, and I hope the U. of Texas will be one. The Geology Foundation and other parts of UT should whip the imaginations of the geologists to discover methane and develop leak-proof CO2 sequestration industries and the petrochemists to make more efficient processes suitable for plants two orders of magnitude larger than present fertilizer plants. Like the jumbo jets that carry the majority of passenger kilometers, compact ultra-powerful ZEPPs could be the workhorses of the energy system in the middle of the next century.
The Continental SuperGrid
Still, energy’s history will not end with natural gas. The completion of decarbonization ultimately depends on the production and use of pure hydrogen, already popular as rocket fuel and in other high-performance market niches. Environmentally, hydrogen is the immaterial material; its combustion yields only water vapor and energy. The hydrogen, of course, must eventually come from splitting water—not from cooking a hydrocarbon source. The energy to make the hydrogen must also be carbon-free. According to the historical trend in decarbonization, large-scale production of carbon-free hydrogen should begin about the year 2020.
Among the alternatives, including solar and photovoltaic routes, nuclear energy fits the context best. I am old enough to have been impressed by schoolbooks of the 1960s that asserted that the splitting and fusing of atoms was a giant step, akin to harnessing fire and starting to farm. We should persist in peacefully applying Albert Einstein’s revolutionary equations. It seems reasonable that understanding how to use nuclear power, and its acceptance, will take a century and more. Still, fission is a contrived and extravagant way to boil water if steam is required only about half of each day to make electricity.
Nuclear energy’s special potential is as an abundant source of electricity for electrolysis and high-temperature heat for water splitting while the cities sleep. Nuclear plants could nightly make H2 on the scale needed to meet the demand of billions of consumers. Windmills and other solar technologies cannot power modern people by the billions. Reactors that produce hydrogen could be situated far from population concentrations and pipe their main product to consumers.
Here let me introduce a second Texas-size idea, the continental SuperGrid to deliver electricity and hydrogen in an integrated energy pipeline. Specifically, the SuperGrid would use a high-capacity, superconducting power transmission cable cooled with liquid hydrogen produced by advanced nuclear plants. The SuperGrid would serve as both a distribution and a storage system for hydrogen, with hydrogen ultimately used in fuel cell vehicles and generators or refreshed internal combustion engines.
By continental, I mean coast-to-coast, indeed all of North America, making one integrated market for electricity. Continental SuperGrids should thrive on other continents, of course, but as an American I hope North America builds first and dominates the market for these systems, which in rough terms might cost $1 trillion, or $10 billion per year for 100 years. The continental scale allows much greater efficiency in the electric power system, flattening the electricity load curve which still follows the sun. Superconductivity solves the problem of power line losses. By high capacity, I mean 40-80 gigawatt (GW).
The fundamental design is for liquid hydrogen to be pumped through the center of an evacuated energy pipe, both to cool the surrounding superconducting cable and to serve as an interstate pipeline for the hydrogen-electricity energy economy [Figure 4]. The cable would carry direct current and might look either like a spine or a ring nearing many of North America’s large cities. Power converters would connect the direct current SuperGrid at various points to existing, high-voltage alternating current transmission substations.
Initially some forty 100-km long sections of the joint cable/pipeline might be joined by nuclear plants of a few GW supplying to the SuperGrid both electricity and hydrogen. High-temperature, gas-cooled reactors promise a particularly high-efficiency and scalable route to combined power and hydrogen production. Nuclear power fits with the SuperGrid because of its low cost of fuel/kwhr and its operational reliability at a constant power level. The hydrogen storage capacity of the SuperGrid, combined with fuel cells, may allow electricity networks to shift to a delivery system more like oil and gas, away from the present, costly, instant matching of supply to demand.
For safety, security, and aesthetics, let’s put the entire system, including cables and power plants, underground. I mentioned earlier that tunneling has a future even if coal mining does not. The decision to build underground critically determines the cost of the SuperGrid. But, benefits include reduced vulnerability to attack by human or other nature, public acceptance by lessening right-of-way disputes, reduced surface congestion, and real and perceived reduced exposure to real or hypothetical accidents and fallout.
An even more evolved concept for the underground corridors combines energy with transport. Sharing the tunnels, magnetically levitated trains in low pressure tubes would run on linear motors of superconducting magnets, speeding from Atlantic to Pacific in 1 hour. I am speaking now of 100 years, but that is our time frame. The maglevs would help spread the infrastructure cost over multiple uses.
As with ZEPPS, magic words for the SuperGrid are hydrogen, superconductivity, zero emissions, and small ecological footprint, to which we add energy storage, security, and reliability.
Conclusion
Evolution is a series of replacements. Replacements also mark the evolution of the energy system. Between about 1910 and 1930 cars replaced horses in the United States. Earlier steam engines had replaced water wheels and later electric drives replaced steam engines. These replacements required about 50 years in the marketplace. It required about the same amount of time for railways to replace canals as the lead mode of transport and longer for roads to overtake railways and for air to overtake roads.
Decarbonization is a series of replacements. Considering primary sources of energy, we find that coal replaced wood and hay, and oil in turn beat coal for the lead position in the world power game. Now natural gas is preparing to overtake oil. The so-called oil companies know it and invest accordingly. We must favor natural gas strongly everywhere and prepare the way for hydrogen, which is a yet better gas.
Importantly, the superior performance of the technology or product fits a larger market. Hydrogen and electricity can cleanly power a hundred megacities.
The global energy system has been evolving toward hydrogen but perhaps not fast enough, especially for those most anxious about climate change. With business as usual, the decarbonization of the energy system will require a century or more. To assuage social anxiety about possible climate change, we should start building ZEPPs, which will pay anyway because of their efficiency.
When increasing spatial density of energy consumption drives the system, we must match it with economies of scale in production and distribution. The coming world of ten billion people needs jumbo jets as the backbone of the energy system, not 2-seater Piper Cubs. Of course, the little planes play crucial roles in the capillary ends of the system and in providing back-up and flexibility. Most effort on the energy system the last couple of decades has been retouching here and there.
Now is the time to think and act big again. ZEPPs and the SuperGrid will bring riches to companies and nations and glory to engineers and scientists and the institutions that nurture them, such as the Geology Foundation and the Jackson School. Let’s commit now to the Texas-size ideas that will complete the grand and worthy challenge of decarbonization.
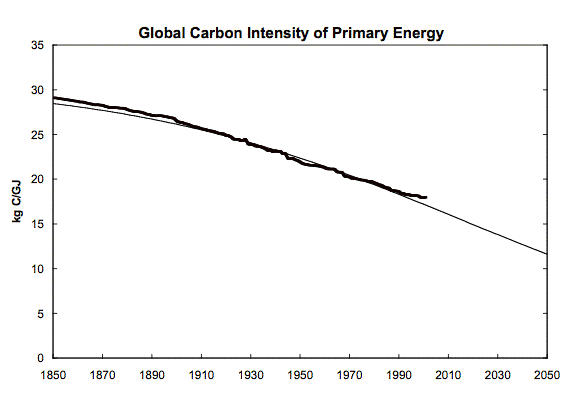
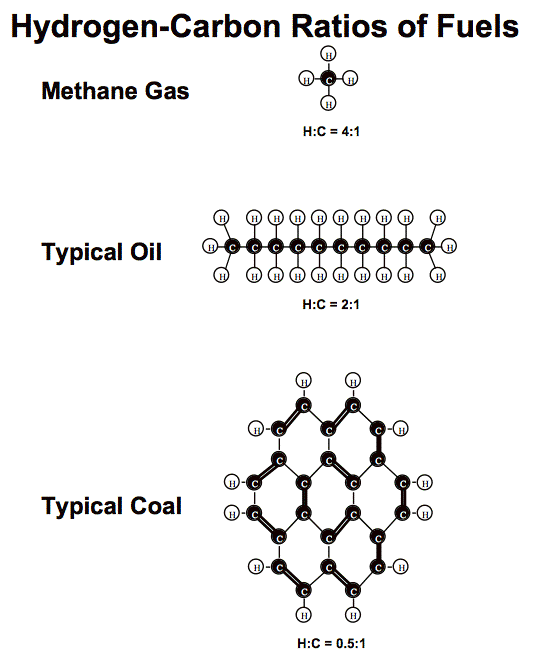
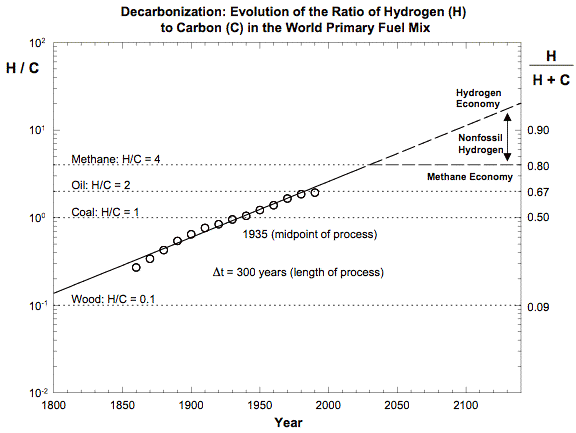
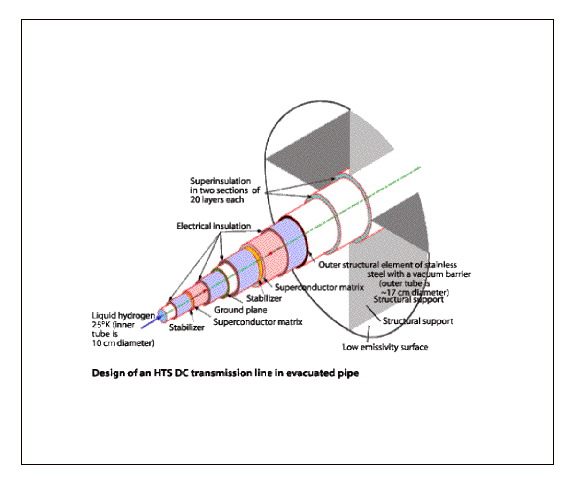
Acknowledgements: Thanks to Cesare Marchetti, Perrin Meyer, Chauncey Starr, and Paul Waggoner.
This talk draws from:
Report of the National Energy Supergrid Workshop, 6-8 November 2002, Palo Alto CA, Thomas Overbye and Chauncey Starr, convenors
Supergrid Sparks Interest, Taylor Moore, EPRI Journal, November 2002, Microsoft Word – SuperGrid Concept Sparks Interest.doc (rockefeller.edu)
Some Ways to Lessen Worries about Climate Change Jesse H. Ausubel, Electricity Journal 14(1):24-33, 2001. https://phe.rockefeller.edu/Lessen_Worries/
Where is Energy Going? Jesse H. Ausubel, The Industrial Physicist 6(1): 16-19, 2000 (February). https://phe.rockefeller.edu/IndustrialPhysicistWhere/
Five Worthy Ways to Spend Large Amounts of Money for Research on Environment and Resources, Jesse H. Ausubel, The Bridge 29(3):4-16, Fall 1999. https://phe.rockefeller.edu/five_worthy_ways/
Resources and Environment in the 21st Century: Seeing Past the Phantoms, Jesse H. Ausubel, World Energy Council Journal, pp. 8-16, July 1998. https://phe.rockefeller.edu/phantoms/
Jesse H. Ausubel is director of the Program for the Human Environment at The Rockefeller University in New York