Citation: Pollution Prevention Review 11 (3): 77–82 2001
Which worries about nitrogen fertilizer are well-founded, and where is the leverage to help? History does not justify worries about exponential increase in use or widespread rising deposition from the atmosphere. Nevertheless, nitrogen leaching and water pollution justify conservation. We analyse four scenarios for fertilizer use to the year 2070. If farmers sustain both their lifting of yields and increasing efficiency of nitrogen use, they can feed future world population better, while freeing the area of ten Iowas for nature and eliminating excess nitrogen.
Belief in an exponential rise of synthetic nitrogen (N) fertilizer use evokes fear of global nitrogen overload (Vitousek et al. 1997; Moffat 1998). Belief that a tandem widespread rise in nitrogen deposition from the air is diminishing biodiversity intensifies the fear of overload. In the background looms the fear that more numerous, richer humans will demand that farmers grow their food with large multiples of today’s nitrogen, and thus push Nature aside.
Meanwhile, in recent newspaper articles, readers learn paradoxically of weak agricultural markets: European farmers demonstrate with their tractors before ministers in Brussels. American farmers invite hunters to shoot their pigs. Manufacturers’ capacity to make fertilizer exceeds demand.
Which fears are well-founded, and where is the leverage to help? This article briefly considers these issues, and suggests some answers.
Keywords: agriculture, nitrogen fertilizer, land use
Areas of Research: Forests, Farms and Materials
Nitrogen Fertilizer
In 1931, Karl Bosch received the Nobel Prize for making nitrogen fixation practical. Like many new technologies, synthetic nitrogen fertilizer enjoyed strong growth, from 1.3 Tg nitrogen in 1930, to 83 Tg in 1998. (One Tg equals a trillion grams or a million metric tons. These data refer to the fertilizer year 1 July-30 June. Thus, for example, 1998 data are for 1998-99.) By “synthetic nitrogen fertilizer,” we mean commercial product, with almost all the nitrogen fixed by the Haber-Bosch process — in contrast to manure, guano, oil meals, and packing-house waste.
Is the use of nitrogen fertilizer growing exponentially? No. A constant percentage increase accelerates a curve exponentially. This is not what is happening with nitrogen fertilizer.
After an explosive annual growth of 14% during the period 1945-56, global growth of nitrogen fertilizer use slowed to 8% as 1970 approached. Growth then slowed further to 5% yearly during 1976-85, and to less than 1% during 1986-98. In Europe and the United States, which adopted the new technology early, growth slowed even sooner. Since 1976, use in Europe and the U.S. has grown 1% or less yearly, and sometimes has actually fallen.
Like numerous products that saturate their market, nitrogen fertilizer fits not an ever-rising exponential growth pattern, but an S-shaped logistic one (Frink et al. 1999).
Nitrogen Deposition
The nitrogen falling from the atmosphere, mostly as NO3-nitrogen and NH4-N (ammonium-N), can be measured by simply collecting it in the open as “bulk deposition.”
“Wet deposition” is collected only during precipitation. “Throughfall” is nitrogen deposited on forest canopies and eventually falling or washing down in precipitation. Nineteenth century agronomists, who were concerned about deposition on crops, collected bulk deposition. More recently, scientists concerned about acid rain (caused by sulfur and nitrogen) collected wet deposition, and ecologists concerned about accumulation of nitrogen in forests collected throughfall beneath trees.
In order to understand nitrogen deposition, we must compare it with some scale. Synthetic fertilizer use has increased approximately 100 fold. Concurrently, worldwide NO3-nitrogen from high temperature combustion in car motors and power plants has risen 10-fold to about 20 Tg.
Farmers increase crop yields with 100 to 200 kg nitrogen ha-1 (kilograms per hectare). For prevention of eutrophication and 95% safety, Europe has established incremental critical loads of 3-10 kg ha-1 (with standards of less than 3 and more than 10 in some places) (Posch et al. 1997).
In infertile soil made responsive by fertilization with other nutrients, increments as little as 10 kg, but sometimes not less than 54 kg, nitrogen ha-1 decreased biodiversity (Tilman 1987). Early in the 20th century, bulk deposition in Europe and the U.S. was 4 to 7 kg ha -1.
Anyone smelling a barnyard knows local deposition of NH4-N can be high. In the Netherlands, a local throughfall of 100 kg ha-1 attributed to animals will affect vegetation (Ivens, 1990). The manure km-2 in the Netherlands is five times that in the quintessential U.S. agricultural state of Iowa. Locally, where animals are concentrated, throughfall can approach farmers’ fertilizer rates.
However, deciding whether widespread, rather than local, increase of nitrogen deposition accompanied the use of more nitrogen fertilizer requires measurements spanning decades at several places. For a comparison that offers data over a period as long as the growth of fertilizer use, we must turn to the simple, robust measure of bulk deposition. In Rothamsted, England, annual deposition rose a total of about 1 kg ha-1 between 1888 and 1966. Bulk deposition of 8.7 kg (estimated from wet deposition during 1987-96 at nearby Woburn) confirms an increase, perhaps 5 kg ha-1 during the century.
In northern Netherlands, bulk deposition increased about 7 kg from 6.7 kg ha-1 at Groningen during 1908-10 to 14.5 kg at nearby Kollumerwaard during 1994. In Sweden, it changed little from the 5.1 kg ha-1 at Flahult in 1909 to 7.1 kg in 1996-97 at three nearby stations (Frink et al. 1999).
At Geneva and at Ithaca, New York, during the first quarter of the 20th century, annual bulk deposition ranged from 4 to 8 kg ha-1. About 6 kg was also deposited at Mays Point and Huntington Forest, New York, during 1965-80 and also at Hubbard Brook, New Hampshire, during 1972-92 (Frink et al. 1999). In the northeastern United States during the 20th century, bulk deposition changed little.
One should not be surprised that nitrogen fertilizer use and widespread increase of nitrogen deposition did not rise in tandem. Except for escape of some ammonia fertilizer and dust and a little NO2, no direct path puts fertilizer nitrogen in the air for deposit. Also, even 80 Tg fertilizer nitrogen plus 20 Tg NO3-nitrogen from high temperature combustion is insufficient to increase widespread deposition significantly; in the impossible case of all 100 Tg being deposited, it would average only about 2 kg ha-1 on Earth. The low concentration of nitrogen in Greenland precipitation (Mayewski et al. 1990) corresponds to deposition far less than that.
Given the moderate likely rise in nitrogen use that we now project, prospects seem good for any future increase in deposition to continue making the minor contribution to plant growth that Nadelhoffer et al. (1999) have calculated is now being made. This should pose little hazard to biodiversity.
Prospects to 2070
After overcoming worries about exponential increase and widespread rising deposition, we still come to the lurking fear of burgeoning, richer humans eventually demanding farmers smother Nature with multiples of today’s nitrogen in order to grow more food. Although nitrogen from fertilizer can scarcely increase widespread atmospheric deposition, its leaching and pollution of water justify conservation.
Foreseeing what humanity will eventually demand requires integrating forces that drive fertilization (Frink et al. 1999). The change of fertilizer use (see Exhibit 1) is the sum of changes in four forces: population, GDP/capita, crop production/GDP, and the Ratio of nitrogen in fertilizer to nitrogen in crops, which we capitalize to “Ratio.” (We neglect the small change over time in the nitrogen composition of the crop mix.) To foresee prospects to 2070, and a population of roughly 10 billion people, we assume a population growing at 0.8% and GDP per capita at 1.8%, to lift their sum, the total GDP, to 2.6% per year.
Although wealthy people eat better than the poor do, they do not eat more in fixed proportion to GDP. Hence, the declining ratio of crops to overall GDP has mirrored the rise of world GDP. Whereas the GDP/Cap data in Exhibit 1 are above the zero change line, the Crop/GDP are below the zero change line, signifying a falling ratio of crops to GDP. Expecting that the world economy will continue to favor computer chips over potato chips, we project a long decline of 1.0%/year in crop/GDP. The sum of GDP per capita rising 1.8%, and crop/GDP falling 1.0%, still raises the crop for all persons by 2070 to the level that rich countries enjoy now.
Farmers control the fertilizer per crop as they economize inputs. Globally, the Ratio of fertilizer nitrogen to crop nitrogen recently plummeted 2.0%/year. In the United States, it fell earlier, and since the 1970s has fallen some 1.0%/year. For the long pull, a decline of 0.5%/year seems reasonable as farmers continue lifting yields faster than their nitrogen fertilizer use grows. The sum of the changing forces (0.8 + 1.8 – 1.0 – 0.5%/year) would raise world nitrogen fertilizer use 1.1%/year to 2.4 times the 1990 use by 2070.
Concern for sparing only fertilizer would be myopic. We integrate with the saving of fertilizer the sparing of land, which is preeminent for sparing Nature. Accordingly, we project cropland taken as well as fertilizer used (see Exhibit 2).
As a baseline for prospects to 2070, consider the situation in 1990, when 79 Tg fertilizer and 11% of world land yielded the caloric equivalent of 1,900 kg ha-1. The 150% Ratio of fertilizer nitrogen to crop nitrogen shows that nitrogen from fertilizer, plus that from legumes and manure, far exceeds nitrogen incorporated into crops, indicating an opportunity for conservation.
Our first projection for 2070 (and assuming a population of ten billion people) is a situation in which farming has stagnated at 1990 levels in terms of yields and Ratio. The consequent 284 Tg fertilizer nitrogen, or 3.6 times 1990 use, exceeds the 2.4 times projected above because the Ratio remains 150%. Under this projection, cropland has expanded because population and crop per capita has grown, while yield has stagnated. A scenario assuming use of 284 Tg nitrogen, and 38% of the land, offers the scary specter of population and wealth eventually demanding that farmers push Nature aside and smother the land in fertilizer.
Researchers can forestall that specter becoming reality with at least two levers: Substitute nitrogen already on farms for nitrogen fertilizer, and raise yields, thus lowering the Ratio of fertilizer nitrogen to crop nitrogen.
Farms fix nitrogen in legumes and collect nitrogen in manure. Although so-called alternative agriculture features nitrogen fixed by legumes, legumes take land and devour natural habitat. Manure is another matter. The estimated 80 Tg nitrogen in the world’s manure matches the 83 Tg fertilizer nitrogen used now and is significant compared to the projected 284 Tg. Remembering that some manure nitrogen is already captured in crops, but that animals may multiply by 2070, one can envision 50 Tg of manure nitrogen substituting for fertilizer nitrogen, decreasing the fertilizer-nitrogen-to-crop-nitrogen Ratio to 124% with crops still using 38% of the land.
The ancient challenge of saving and storing nitrogen without odor continues. Concentration of animals has focused attention on odor and increased the distances for hauling manure to fields. It has also changed the goal from conserving nitrogen to denitrifying it and controlling odor. A challenge for pollution prevention is replacing the negative tasks of regulating odor and returning N2 to the air with the positive task of discovering profitable and practical ways to move more of the dilute nitrogen in manure into crops.
Conserving fertilizer can also lower the Ratio of fertilizer nitrogen to crop nitrogen. Conservation includes testing soil nitrogen and adjusting application to each site by precision farming and splitting applications during the season to avoid leaching. Because yields divide the Ratio, higher yields can lower the Ratio and spare land, too. Increasing yields to lower the Ratio involves breeding better crop varieties, plus removing limitations such as other nutrients, water, and pests so that crops can exploit the nitrogen provided.
While words about lowering the Ratio of fertilizer nitrogen to crop nitrogen could be mere anecdotes, statistics show farmers are in fact doing it (see Exhibit 1). During the 1980s and 1990s the worldwide Ratio fell. In the United States since the 1970s, the Ratio of fertilizer nitrogen to crop nitrogen fell about 1%/year. With regard to a specific locale and crop, the Ratio for Indiana, Iowa, and Nebraska corn fell 1% to 3% per year during 1980-96 (Frink et al. 1999). Farmers lifting the world average yield of corn, rice, soybeans, and wheat by an annual average of 1.6 to 2.3% during 1961-2000 demonstrate that higher yields also are not mere anecdotes.
A projection involving slower lifting of yields, but conserved nitrogen, shows that raising yields 1% and lowering the Ratio 0.5%/year would take 19% of land, while lowering nitrogen fertilizer use to 192 Tg. This is the 2.4-fold increase we deem probable.
With sustained lifting of yields and conserved nitrogen, raising yields 2% and still lowering the Ratio of fertilizer nitrogen to crop nitrogen would require the same 192 Tg of nitrogen, but would actually shrink cropland 144 million hectares, which is about half the area of India, or ten Iowas.
Today, fertilizer manufacturers have idle capacity. This, combined with weak agricultural markets in Europe and the U.S., reveal how groundless are fears that fertilizer use will soon explode. There is little prospect of a widespread downpour of nitrogen from the air, or Nature pushed aside to relieve hunger.
Outline for Future Action
For the long run, however, and to relieve “hot spots” of nitrogen deposition, several things need to be done. On the grand scale, regulators need to monitor two simple environmental metrics: land spared from cropland expansion and the Ratio of fertilizer nitrogen to crop nitrogen.
On the local scale, where action is taken, fertilizer manufacturers should offer information on how to make fertilizer go further while farmers, the most hard-pressed actors, struggle to survive. Researchers, the actors with the most opportunity to effect change, should devise affordable ways for farmers to utilize manure (now seen as a nuisance) for higher yielding crops.
Meeting these challenges will temper the use of nitrogen fertilizer, while sparing more land for Nature.
Exhibits
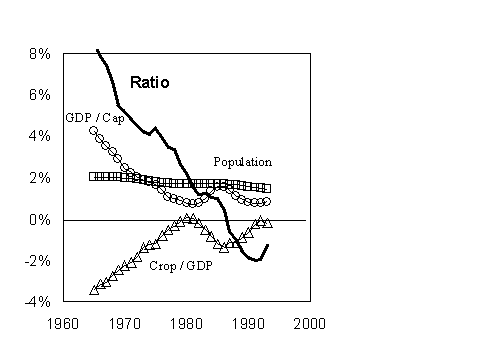
Exhibit 1. Annual Global Changes in Population, GDP per Capita, Crop Production per GDP, and the Ratio of Synthetic Fertilizer Nitrogen to Crop Nitrogen.
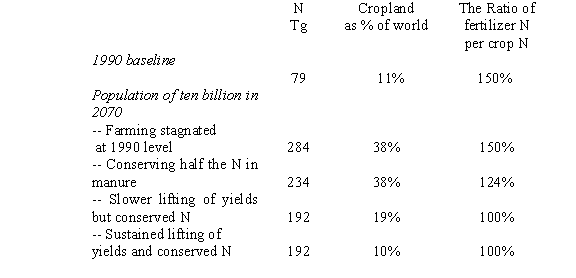
Exhibit 2. Prospects to 2070.
References
Ivens, W.P.M.F. (1990). Atmospheric Deposition onto Forests. Analysis of the Deposition Variability by Means of Throughfall Measurements. Nederlandse Geographische Studies 118, 151 p.
Mayewski, P.A., et al. (1990). An Ice-Core Record of Atmospheric Response to Anthropogenic Sulphate and Nitrate. Nature 346:554-556
Moffat, A.S. (1998). Global Nitrogen Overload Problem Grows Critical. Science 279:988.
Nadelhoffer, K.J., et al. (1999). Nitrogen Deposition Makes a Minor Contribution to Carbon Sequestration in Temperate Forests. Nature 398:145-148.
Posch, M., Hettelingh, J.-P., de Smet, P.A.M., & Downing, R.J. (1997). Calculation and Mapping of Critical Thresholds in Europe. RIVM Report 259101007.
Tilman, D. (1987). Secondary Succession and the Pattern of Plant Dominance along Experimental Nitrogen Gradients. Ecological Monographs 57:189-214.
Vitousek, P.M., et al. (1997). Human Alteration of the Global Nitrogen Cycle: Sources and Consequences. Ecological Applications 7:737-750.
Charles R. Frink (charles.frink@po.state.ct.us) is a soil expert and scientist emeritus at the Connecticut Agricultural Experiment Station in New Haven. Paul E. Waggoner (paul.waggoner@po.state.ct.us) is an agronomist and meteorologist, and past director of the Connecticut Agricultural Experiment Station. Jesse H. Ausubel (ausubel@mail.rockefeller.edu) is an industrial ecologist and director of the Program for the Human Environment at The Rockefeller University in New York City (https://phe.rockefeller.edu).