Area of Research: Energy and Climate
The future environment for the energy business
Does Climate Still Matter?
We may be discovering climate as it becomes less important to well-being. A range of technologies appears to have lessened the vulnerability of human societies to climate variation.
Amidst widespread agreement that the planet is committed to at least some climatic change induced by human activity, there is growing pressure to “slow the greenhouse express” (ref. 1). Here I examine climate through the lens of technology and innovation, to clarify what adaptations have succeeded and the trends in vulnerability to climate. I also examine whether the greenhouse effect by itself will call into play new technologies, or whether the evolution of technology will largely be ‘business as usual’ regardless of climate change. Finally, I identify some ways that government may assist adaptation.
My focus is on adaptability of human systems, including agriculture. Adaptability of ecosystems and the ethics of human behaviour that brings about large scale transformations of the Earth must also be considered in balancing responses to the greenhouse issue.
What are often labeled adaptive measures are themselves the impacts of climatic change. Innumerable adaptations in food, clothing and shelter are responses to the spatial and temporal variability of climate. Humans do not wait guilelessly to receive an impact, bear the loss, then respond with an adaptation. Rather they attempt to anticipate and forstall problems.
Innovations
Technical innovations relevant to climate and their diffusion occur in all societies and sectors and in many forms. Technology includes both hardware (for example, materials, physical structures, devices and machines) and software (rules and recipes for behaviour).
Illustrative innovations of hardware are cisterns and dams to store water, tractors to speed rapid harvests, and new crop cultivars to reduce susceptibility to drought. Perhaps less obvious, but of great importance for adaptation to climate, are information technologies. In the United States, during several years in the 1980s sales of information technologies to the agricultural sector were comparable to sales of farm equipment. The long history of software innovations includes tide tables, irrigation scheduling, and weather forecasts. Along with the readily classified hardware and software are climate related behavioural, social and institutional innovations, such as agricultural credit banks, national parks, green political parties and flood insurance.
Software and social innovations are al most always indispensable for the technology of new hardware. Major innovations, in transportation for example, are in fact clusters of innovations involving not only new materials and physical processes but also new forms of social organization, including financing and management. Transportation systems exemplify technology that has been important in adaptation to climate through expanding the availability of food from a local to a global scale. Reliance on food from afar not only diversifies diet, but also spreads production risks across more climatic zones.
Early communities drew the bulk of their food from small areas. One of the earliest city states, Uruk in Mesopotamia, probably grew most of its sustenance except for animals within 20 kilometres of the city walls2. Two millennia later, Greece and Rome obtained most of their food from overseas colonies across the Aegean and Mediterranean seas3. At its height, Rome acquired 200,000 tons of grain annually for its one million inhabitants, most of it shipped from Africa, Sardinia and Sicily. What marine transportation did in the classical world, the steam locomotive did in the nineteenth century, halving the cost of land transport. The railroads penetrated the great land masses of North America and Australasia. Their operations were little affected by climate, topography or other local conditions. Great parts of the new worlds were dedicated to cultivation of single crops to supply world markets and to smooth availability through the year.
Technological inventions and innovations that have had roles in human ability to adapt to climate over the last 100 years or so range widely4: food preservatives (1873) to over come problems of seasonal food production; light bulbs (1879) to make work safe and effective indoors; aluminum (1887) and other structural materials to resist environmental deterioration; refrigeration (1895) and air conditioning (1902, 1906) to facilitate activity in hot seasons and locations; automobiles (1890s) to provide personal transportation that is much less sensitive to climate than horses or pedal bicycles; mechanical wind shield wipers (1916) to see in the rain; anti freeze (ethylene glycol) (1929) to safeguard motors in winter; frozen food (first sales, 1930) to diversify diet among regions and seasons; radio-beam navigation (1934) to fly in poor visibility; and weather (1960) and Earth resources (1972) satellites for analysis and forecasts of weather and climate.
In many respects we seem to be ‘climate proofing’ society, making ourselves less subject to natural phenomena. For centuries and millennia we relied mainly on behavioural and social adaptation. We took siestas when the sun was high and sought refuge in hill stations in the monsoon season. Large pastoral and nomadic populations followed the seasonal availability of resources and avoided climatic stresses. Much of the planet remained seasonally or entirely uninhabitable for climatic reasons. With current technology many people can live in virtually any climate that now exists. Modern water supply and heating and ventilation systems, along with medicines (for example, quinine and vaccines) and public health measures, have enabled large populations to inhabit formerly uninhabitable regions. By 1980 the population in semiarid, desert and mountain regions had passed 35 million or 15 per cent of the US population5. Lacking modern technology, these zones accomodated less than one per cent of the population in 1860 and six per cent in 1920.
Preferences
The ability to colonize almost the entire planet is due to the human ability to carry with us that particular range of environment in which we can survive and prosper6. In wealthier societies, preferences are shifting toward hot and dry climates that were forbid ding a century ago. Evidence of lessening human vulnerability is also found in health. For example, a flattening of monthly rates of total mortality in Japan between 1899 and 1973 is explained partly by diminution of the previous, climatically driven seasonal peaks7.
Production can now proceed more or less continuously in severe environments. For example, North Sea offshore oil platforms operate 24 hours per day, 365 days per year. At a price, services, such as aviation, are now available at almost all times and places. Aviation began as a system that was extremely sensitive to weather and is increasingly less so, due to expanded range; avionics, radar and guidance systems; understanding of thunderstorms, wind shear, and other weather phenomena; and changes in construction materials. Crops and livestock can now be produced ‘indoors’ protected from the elements. In some cases, alternatives to outdoor production are so advantageous that a crop is displaced. Originally spurred by the need for supplies in wartime, synthetic rubber from petrochemical feedstocks, which is not subject to the vagaries of pests, droughts and floods, and other risks out doors, has overwhelmed natural rubber from trees. In 1990, ten per cent of US fish production was in the controlled environment of fish farms8, up from one per cent in 1980 and projected to reach 20 per cent in 2000.
Consumption is also insulating itself from environment. Inside most shopping malls, for example, only fashions or decorations signal the season. Sports are increasingly played in domed stadiums isolated from the weather. In affluent societies, winter vacations in warm climates have become a popular adaptation to escape climatic impacts.
Forecasting is itself a key technology that reduces vulnerability to weather and hence climate. Forecasts can help accommodate peak loads in electric power systems during heat waves. Improved forecasting, in conjunction with increased incomes and better transportation, has also enabled more people to enjoy recreation in all seasons.
Synchronicity
The decline of ‘synchronicity’, the naturally enforced time regimen of social groups, is a feature of life in advanced societies. In agricultural societies, the rhythm of life is strongly determined and coordinated for al most all by the seasons and the associated demands for labour in the field. In advanced economies, where both production and consumption may proceed almost continuously and only about five per cent of the population farms, weather and climate no longer control schedules. The fact that the peak season for holidays in advanced societies is the late summer, a peak season for labour in agricultural societies, indicates the transformation that has taken place.
In the late 1970s and early 1980s a group of US researchers explored the ‘lessening’ hypothesis of climate impacts9, which states that persistent and adaptive societies, through their technological and social organization, lessen the impacts of recurrent climatic fluctuations of similar magnitude upon the directly susceptible population and indirectly lessen the impacts on the entire society. In the cases studied, substantial evidence was found to support the hypothesis of lessening impacts. For example, in the US Great Plains, the most severe disruptions to livelihood and health occurred during the earliest periods, when incidences of malnutrition and starvation were recorded. Investigation of the more recent periods showed much smaller impacts for comparable drought stress, because of a variety of adjustments and strategies, including more extensive and effective anticipatory action.
An alternative to the lessening hypothesis is that increasingly elaborate technical and social systems insulate us from the adverse effects of recurrent climatic fluctuation at the cost of increased vulnerability to catastrophe from less frequent natural and social perturbations. In a global economy, such vulnerability might be devolved or shared ever more widely. Presumably this vulnerability to catastrophe, surprise or nonlinear effects is what worries many about the greenhouse effect. But the evidence seems to weigh against the suggestion that technology, lifestyles and other forces are creating a more ‘brittle’ system in the face of climate change.
Society appears to proceed along ‘technological trajectories’ that enable, for example, more travel, more financial transactions, and more messages. The succession of technologies that make possible this increased activity appears to diminish in sensitivity to climate. Although it was usually true that neither rain nor sleet stayed the American postman from his rounds, no system of postmen could be as faithful as the modern telecommunications system that now carries a much larger share of messages than the old system of letters. Similarly, a system of energy from wood and hay was more climatically sensitive than one reliant on oil and natural gas. Water and wind power are, of course, more sensitive to climate. In the late eleventh century, the areas under Norman rule in England had about one water mill for every 50 households10, providing power to grind grains, work metal, weave textiles and cut wood. In 1694 France had 80,000 flour mills, 15,000 industrial mills, and 500 iron and metallurgical works, altogether almost 100,000 facilities powered by wind and water. Although such an industrial infra structure is tightly adapted using climatic resources, it is also vulnerable to climate variability and change.
The trend toward less climatic vulnerability also exists in transportation. Well into the nineteenth century, sailing vessels were the preferred long-distance transport and frequently becalmed. World steamship tonnage exceeded sailing tonnage only in 1893. Although coal cost more than wind, steam ships rapidly became cheaper as well as faster than sailing ships, because their schedules were more regular and avoided the circuitous routes required by sailing vessels. Transport underground through tunnels by high-speed magnetically levitated trains is already on the drawing boards in Japan; such systems would be less sensitive to climate than the surface and air systems now in use.
Climate is only one of several factors that have driven the evolution of systems of communications, transport and energy. It is probably secondary. But vulnerability to climate and other environmental forces may be a good proxy for quality and reliability of service. To the extent that the systems evolve in the direction of higher quality and reliability, these trajectories of development may also decrease vulnerability of major systems to climatic change. It would be useful to identify exceptions to this pattern, should they exist.
As incomes depend less on activities out of doors, societies become less vulnerable to climate. The trend in all developed countries since the industrial revolution began is away from employment outdoors (Fig. 1) and to ward employment in the service sector, most of which is in climate-controlled office buildings and shops. With a lag of 50-100 years the same trend is found in less developed countries, where 4050 per cent of the population is now engaged in agriculture. if the current trend continues, this fraction should diminish to 20-25 per cent by 2050.
What are the times characteristic of technical innovation and diffusion of technologies in relation to the time of human induced climatic change? Taking account of both C02 and non-CO2 greenhouse gases, major climatic shifts are expected during 40-50 years (ref. 11). A retrospect on technology during the past century suggests the extent of change during the decades to come: in 1890 there was little farming in California and Australia, and key technologies did not yet exist or were not widely applied to impound and transport water, or to transport and store agricultural products promptly; in 1903 the Wright Brothers flew 59 seconds at Kitty Hawk, North Carolina, whereas in 1990 in the United States, over 500 million air passengers flew around 450 x 109 miles12; commercial transatlantic aviation, relying on the jet engine, superseded travel by ocean liners about 1960; tourism has been extended to Antarctica, and scientific bases there are occupied year round, despite the fact that it was only in 1911-1912 that Roald Amundsen reached the South Pole and Robert Scott died there; penicillin, the first important antibiotic, was discovered by Alexander Fleming in 1928, and large-scale production began only as recently as 1943; nuclear power for electricity generation first came into use in the late 1950s, but within about 30 years it was able to provide over 70 per cent of France’s electric power; until 1965 no satellites were used for any routine application, whereas satellite systems now girdle the Earth, watching for storms, relaying communications, and helping ships and planes navigate anywhere on the globe; and finally, although the microprocessor was only introduced in 1971 and the personal computer appeared about 1977, Americans are now using over 50 million personal computers.
During the period in question and with or without climatic change, technology will transform the way people live. Food, energy, transportation and all the other systems that support human life and the economy will be changed by technologies that can be glimpsed now, such as genetic engineering, fusion, superconductivity and desalination, as well as by technologies yet to be easily pictured. Fifty to a hundred years will allow the replacement of most major technological systems. Indeed, 50 years are enough time to turnover almost the entire capital stock of the society. About two-thirds of capital stock is usually in machines and equipment and about one-third in buildings and other structures. In Japan, the average renewal period for capital stock in business, the time it takes for machinery and equipment in an industry to be almost completely replaced, ranges from about 22 years in the textile industry down to ten years or less in such fast-moving industries as telecommunications and electrical machinery (see Table). In agriculture the estimated life span of cultivars in the United States is seven years for maize, eight for sorghum and cotton, and nine for wheat and soybeans13, and most experts believe the life span of cultivars will grow shorter, Relative to greenhouse warming, turnover is also fast for nonmachinery capital stock, which includes buildings, pipelines, and so forth. According to recent surveys in the Federal Republic of Germany in 1985 some 60 per cent of the stock is less than 20 years old and in the Soviet Union some 80 per cent is less than 20 years old (Fig. 2).
At first such figures may surprise us. But some reflection about the built environment relieves the surprise. Consider the office space in a modem city. Most of the space is in buildings completed in the last 20-30 years. These new buildings are filled with new equipment, for example, new telephone systems. Indeed, even older buildings are filled with modem equipment that did not exist 15 or 20 years ago. The same is true for super markets, restaurants and other stores. A large fraction of residential housing is similarly young and, in turn, filled largely with new domestic appliances of all kinds. If societies grow at a rate of 2-3 per cent per year, as the industrialized societies have for the past 150 years, then half of all capital stock will always be younger than 30 years old.
Probably the systems that take longest to build are infrastructures. Even these are constructed in less than a century14. Many infra structure systems are (or should be) continuously reconstructed. For example, roads are repaved every 5-15 years, depending on use. The 7,000-kilometre canal system of the United States was almost entirely built in the 30 years between 1820 and 1850. More than 90 per cent of the 300,000-mile US railway network was laid in the 65 years between 1855 and 1920. The paving of virtually the entire six million-kilometre surface road system of the United States was accomplished between 1920 and 1985. The US interstate highway system was completed in about 30 years from the time that it was announced by President Eisenhower. It is interesting to consider whether climatic change could re quire any public works on this scale; coastal protection and interbasin water transfer would seem the most likely candidates.
Because capital stock continuously turns over on a time scale of a few decades, it will be possible to put in place much technology that is adjusted to a changing climate. This ran be done without extraordinary measures given reasonably accurate information about the future. For the shortest lifetimes, even accurate information about the present climate will do.
That perhaps 90 per cent of the global capital stock in the year 2040 will be bat after 1990 does not diminish the significance of some long-lived structures. Action may be necessary to protect cities such as Venire, where preservation of historic buildings is the goal. In such cases, the process of replacement is not relevant.
The adaptations for long-term climatic change will probably mostly be the same as for other climate variation. The technologies, small and large, that buffer human activity over the long-term will be the same ones that mollify the difference between day time and nighttime temperatures, protect against normal variability between days, shield from storms and hail, adjust to the sea sons, and adapt to the wide range of climates where people already live.
No one has yet presented a radical innovation uniquely adaptive for the greenhouse effect. The main innovations directed at the greenhouse effect are so far organizational, in particular research groups in universities and government and assessment groups, such as the Intergovernmental Panel on Climate Change (IPCC). If the future green house climate in any place will consist of climates that already exist somewhere on Earth, then many of the adaptations may look familiar.
Because the population of the world is imploding into cities, it seems logical that technologies that make cities habitable in un-welcoming climates will be among the technologies that are most important. Houston, Phoenix and Toronto may offer lessons. The trend in such places is toward ever larger en closures of space and passageways connecting them, where workers and shoppers are not subject to the elements. Already during much of the year in such cities few people are seen outside on the streets. Technologies for ‘smarter’ buildings and for more efficient building materials should be adaptive. Cities in developing countries, which are often in difficult climates to begin with and face worsening problems of urban pollution, may well lack the resources to apply such technologies to raise or maintain the quality of life in the face of changing climate.
Until this century much of the human struggle with climate was to keep warm. Because the struggle succeeded, in 1850 the population in Europe, a land of well chronicled and damaging winters, was three times as large as that of Africa and nine times as large as that of Latin America15. Now a main change in adaptation will be emphasis on technologies to stay cool. There is already pressure and success in this direction, population grows in tropical regions and people migrate south in temperate zones. Chemicals for refrigeration that do not exacerbate the greenhouse effect may thus earn a premium, and, of course, low greenhouse gas emission technologies to produce electricity and energy in general.
For water resources, larger-scale control of flows may be the trend. The Thames Barrage and the Netherlands Rhine Delta scheme may exemplify massive hydraulic systems that will be imitated in areas of major coastal populations. Freshwater systems in some regions would also be made more robust by extending networks of supply over wider spaces through interbasin transfer and other strategies. In practical terms, many of the technologies needed may be well-established, for example, tunnelling, pumps and other technologies traditional to civil and mechanical engineering, updated with electronic sensors and other devices for management and control. Technologies for management of water demand will be equally or more attractive in many regions; these would include not only hardware technologies for minimizing leaks, but also software technologies from operations research, as well as economic and other incentives.
In agriculture, with a few notable exceptions, most emerging technologies are expected to reduce substantially the land and water required16. At least in the United States the trends in agricultural technology are in the direction that should be sought in view of climatic change. Almost all technologies that are attractive for agriculture are only more attractive in light of the possibility of climatic change. Specifically, appealing directions for agricultural innovation might include diversification of crop production by varying maturity, heat and drought tolerance, input needs, and end uses; innovation in planting and spacing; collecting and recycling irrigation run-off; soil moisture conservation; better moisture-use efficiency and improved use of plastics and other new materials; resistance to pests and insects; management practices; institutional measures; programmes and facilities to support extreme contingencies; and infrastructure. Many of these are applications of information technologies, as well as biotechnologies and more traditional agricultural and mechanical technologies. It may be possible to design and select plants adapted to higher concentrations of CO2, and other changes in the atmosphere.
Though many innovations helpful in a rapidly changing climate are more likely to come from private enterprise than government, governments ran help in two ways.
One way governments ran aid adaptation is through timely information. One variety is assessments of the issues relating to climatic change. A second important and more operational variety of information is improved weather and climate forecasts. Eventually the climate of the far future will become to morrow’s weather. Information about it is likely to improve the possibility that it will be more resource than hazard.
There has been a gradual, measured improvement in weather forecasting during the past 20 years (ref. 17). In the greenhouse issue, all nations should find strong motivation to improve forecasts and the data and research underlying them. The quality of weather analyses and forecasts in many developing countries, especially in the tropics, is markedly lower than those in developed countries, particularly in the northern hemisphere. Advances in numerical modeling and extension of technology for monitoring in tropical regions can cause substantial improvements.
A third innovation in information relates to markets. The needs are for facilitation of information flows and improvements in rules for markets, in particular, markets for water. In many nations, water is allocated largely through administrative means based on water rights. Water transfers accommodate new patterns of climate, as well as changing farming and urban and industrial growth. They allow water to be used where it is most valuable18. Flexibility of water transfer is important, because the life of water projects is often 50-100 years or more19.
Substantial subsidies for water for irrigation, in particular, lead to prices that en courage inefficiency. ‘Mere is little incentive to conserve. Higher cost of constructing water projects and more demand and competition for water for such uses as preservation of wildlife, recreation, and cities make the issue serious. Several long-term con tracts in the Central Valley of California provide water for only $3.50 per acre-foot, whereas new sources of supply would cost the federal government or the state $200-300 per acre-foot per year for construction alone18. Allowing and encouraging voluntary marketing of the resource among users could help adapt to climate changes and produce economic benefits. Voluntary water transfers could take several forms, including permanent sales, long-term leases, short term leases, and leases contingent on drought.
Although markets may require innovation by government in providing information and rules, there are also traditional ‘hardware’ opportunities for innovation in public works. Government is the primary purchaser, financier and manager of systems of water supply and waste disposal, as well as coastal facilities. These take decades to site and construct and then can last generations. There may be opportunities for government to enhance innovation in infrastructure in light of the possibility of a changing environment.
Conclusion
Technologies are available for adaptation to climate on a spectrum of space, time and cost. Within minutes and for a few dollars one can buy an umbrella for local protection against a shower. Such technologies diffuse rapidly into the society, in a matter of months or years. Larger and more costly innovations, like electric refrigerators, may take 20 or 30 years to become pervasive. At the other end of the spectrum, large systems, like those for water and transportation take several decades or generations to extend themselves fully and may cost tens or hundreds of billions of dollars.
Technological performance has improved throughout human history, and in this century waves of innovation have come ever more rapidly20. In many systems, there have been steady improvements in efficiency of about two per cent per year, so that systems built today, for instance in energy, are about twice as efficient as those built 30 years ago21. Today generating a kilowatt of electricity in a steam plant takes only 15 per cent as much fossil fuel as at the turn of the century. A doubling of overall efficiency of several major systems should be possible just by replacing existing systems with best technologies and practices available today. This, of course, takes capital, and it is not clear that the expected rate of climatic change warrants an acceleration over the rate of change in physical capital stock that is already occurring, as long as the new stock is acquired with the best information about future climate in mind.
The general direction of change in technology and civilization is heartening for those anxious about climatic change. The trend is toward systems that are less vulnerable to climate. It would seem to be sensible to maintain this course and not to revert to reliance on such technologies as sailing ships and water mills that are more sensitive to climate. The highest need is probably to assure the inventive genius, economic power, and administrative competence that make the many technologies useful in adapting to climate available to the most people.
Figures

FIG. 1 Share of the workforce employed in agriculture21.
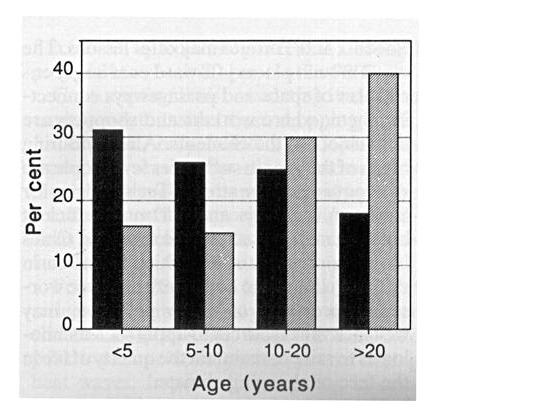
FIG. 2 Age distribution of nonmachinery capital stock in the FRG, data for 1985 (ref. 23) (hatched bars), and in the Soviet Union (solid bars), data as of 1986 (USSR State Committee on Statistics, Statistics on social indicators and capital vintage structure in industry, undated memo, Institute for Social and Economic Statistics, Moscow; courtesy of A. Grübler, Laxenburg, Austria).
Table
CAPITAL STOCK RENEWAL
Industry | Renewal period |
---|---|
All Industries | 13.4 |
Manufacturing (all) | 15.8 |
Electrical machinery | 9.8 |
Transport machinery | 13.2 |
Pulp and paper | 13.7 |
General machinery | 14.2 |
Food stuff | 16.7 |
Steel and non-ferrous metals | 21.1 |
Textiles | 22.5 |
Non-manufacturing (all) | 11.8 |
Service | 8.1 |
Transport and Telecommunications | 10.7 |
Construction | 11.9 |
Finance and insurance | 12.8 |
Distribution | 15.6 |
Real estate | 15.8 |
Electricity, gas and water supply | 15.8 |
CAPITAL STOCK RENEWAL: Average renewal period for capital stock of business corporations in Japan by industrial sector, 1986-87 (ref. 22).
References
1. Nordhaus, W. D. Setting National Priorities. Policy for the Nineties (ed. Aaron, H.) 185-211 (Brookings, Washington, 1990).
2. Adams, R. McC. & Nissen, H. The Uruk Countryside: The Natural Setting of Urban Societies (University of Chicago Press, Chicago, 1972).
3. Newman, L. F. (ed.) Hunger in History: Food Shortage, Poverty, and Deprivation (Blackwell, Oxford, 1990).
4. Desmond, K. Harwin Chronology of Inventions, Innovations, and Discoveries from Pre-History to the Present Day (Constable, London, 1986).
5. Schelling, T. C. in Changing Climate (National Research Council) 449-482 (National Academy Press, Washington, 1983).
6. Escudero, J. C. Climate Impact Assessment (eds Kates, R. W., Ausubel, J. H. & Berberian M.) 251-272 (Wiley, Chichester, 1985).
7. Weihe, W. H. Proceedings of the World Climate Conference 313-368 (World Meteorological Organization, Geneva, 1979).
8. New York Times, 1 August 1990, p. C1.
9. Warrick, R. A. Climatic Constraints and Human Activities (eds Ausubel, J. & Biswas, A. K.) 93-123 (Pergamon, Oxford, 1980).
10. Reynolds, T. S. Sci. Am. 251(1) 122-130 (1984).
11. Intergovernmental Panel on Climate Change Scientific Assessment of Climate Change, Report to IPCC from Working Group 1 (World Meteorological Organization, Geneva, 1990).
12. US Bureau of the Census Statistical Abstract of the United States: 1990
110th edition (US Government Printing Office, Washington, 1990).
13. Duvick, D. N. Econ. Botan. 38, 161-178 (1984).
14. Grübler, A. The Rise and Fall of Infrastructures: Dynamics of Evolution and Technological Change in Transport (Physica, Heidelberg, 1990).
15. McEvedy, C. & Jones, R. Atlas of World Population History (Penguin, New York, 1978).
16. Office of Technology Assessment, Technology, Public Policy, and the Changing Structure of American Agriculture (US Government Printing Office, Washington, 1986).
17. World Meteorological Organization (WMO) The World Weather Watch: 25th Anniversary 1963-1988 (WMO No. 709, Geneva, 1988).
18. Wahl, R. W. Markets for Federal Water: Subsidies, Property Rights, and the Bureau of Reclamation (Resources for the Future, Washington, 1989).
19. Waggoner, P. E. (ed.) Climatic Change and US Water Resources (Wiley, New York, 1989).
20. Mensch, G. Stalemate in Technology (Ballinger, Cambridge, 1979).
21. Nakicenovic, N. & Grübler, A., Technological Progress, Structural Change and Efficient Energy Use: Trends worldwide and in Austria, International Part (International Institute for Applied Systems Analysis, Laxenburg, 1989).
22. Economic Planning Agency Economic Survey of Japan, 1987-1988 (Tokyo, 1989).
23. Statistiches Bundesamt Wirtschaft und Statistik 4/89 (Metzler-Poeschl, Stuttgart, 1989).
Where is Energy Going?
This article appeared in the magazine The Industrial Physicist, published by the American Institute of Physics, in the February 2000 issue.
The essay had appeared in Italian in the special millennial edition of the Italian financial newspaper, Il Sole/24 Ore, on 17 November 1999; also in Italian as Benvenuti nel millennio nucleare, pp.163-168 in Duemila: Verso una societa aperta, M. Moussanet, ed., Il Sole 24 Ore, Milano, 2000.
There was an error in the printed version of Figure 3. Both the figure labeling and caption are wrong. The error has been corrected in the PDF version here and on The Industrial Physicist web site.
Figure 3 should read:
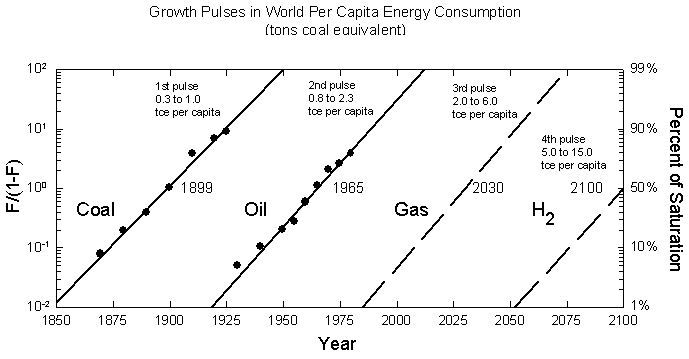
Figure 3. When total world per capita energy consumption (tons coal equivalent) is dissected into a succession of logistic curves, normalized on a scale that renders each S-shaped logistic pulse into a straight line, energy history is a succession of growth pulses evolving around the lead energy commodity of the era. In each era, consumption triples. “F” is the fraction of market share.
To view a PDF on your computer, you can download Adobe Acrobat Reader from Adobe at https://www.adobe.com/.
Economics in the air: an introduction to economic issues of the atmosphere and climate
Energy and Environment: The Light Path
This paper is dedicated to Cesare Marchetti, who lit the path.
Our environmental problem started long ago when the Judeo-Christian God instructed Adam and Eve to go forth and multiply. For 3 million years or so, we tried and failed this assignment and thus lived in balance, more or less, with the rest of creation. The balance was a painful, unsatisfying one for humans, with high mortality, a chancy diet, and life restricted to a narrow range of hospitable climates. When we harnessed fire half a million years ago, the first major step was taken toward solving the problems of the raw, the cold, and the dark; fire would vastly extend human range and food supply. With the invention of agriculture 10,000 years ago, our species finally obtained the means for steady increase.
In 1859 Charles Darwin published the scientific basis for the biblical maxim to multiply. Recall the full title of Darwin’s classic, On the origin of species by means of natural selection or the preservation of favored races in the struggle for life. Darwin’s research followed a century of engineering breakthroughs that made possible the transition from addition to multiplication.
The agricultural story is important for the environment in numerous ways, but one merits emphasis here. The high rates of productivity and labor efficiency, eventually achieved by the mechanization of agriculture, released people from the land and fed the growth of cities. In the nineteenth century, metropolitan London was the largest city with perhaps 10 million people. Today Japan’s Shinkansen Corridor extending from Tokyo to Osaka houses some 80 million. Worldwide the human population is now 55 percent urban. By the time population reaches 10 billion, the urban share may be 70 or 80 percent. We will live in a world of many enormous urban agglomerations.
City size and density are probably the most important factors in determining social complexity and technological evolution. The size of a city, defined as a large group of people connected in daily routines, depends on both population and speed. Higher speed reduces density, keeping population constant, or increases population by extending area at constant density. The size and density enable specialization and bring together people to combine ideas. They provide filters and competition for selection and set the market niche for new ideas and products. The technological paradigms for the world emerge from the high-level metropolises. Their growth and the interactions they have with one another and the hinterland pose the most difficult technical problems of communication, transport, and other needs and focus the resources to address them.
By 1800 or so in England and other early loci of industry, high population density and the slow but steady increase in energy use per capita increased the density of energy consumption. As the British experience demonstrated, if energy consumption per unit of area rises, the energy sources with highereconomies of scale gain an advantage (Marchetti, 1975). Wood and hay, the prevalent energy sources at the start of the nineteenth century, are bulky and awkward to transport and store. Consider if every high-rise resident needed to keep both a half cord of wood at hand for heat and a loft of hay for the Honda. Think of the problems of retail distribution of these goods in cities with land prices of Tokyo, Singapore, Frankfurt, or Paris. Sales of wood in cities today are, of course, limited to an occasional decorative log providing more emotional than physical warmth. Biomass gradually lost the competition with coal to fuel London, Manchester, and other multiplying and concentrating populations, even when wood was abundant.
Coal had a long run at the top of the energy heap. It ruled, notwithstanding its devastating effects on miners’ lungs and lives, the urban air, and the land from which it came; but about 1900 the advantages of an energy system of fluids rather than solids began to be evident. On the privacy of its rails, a locomotive could pull a coal car of equal size to fuel it. Coal-powered automobiles never had much appeal. The weight and volume of the fuel were hard problems, especially for a highly distributed transport system. Every automobile filling station could not have a rail siding to deliver its coal supply nor the acreage to store its coal. Oil had a higher energy density than coal–plus the advantage of flowing through pipelines and into tanks. Systems of tubes and tins can deliver carefully regulated quantities of fuel from the scale of the engine of a motor car to that of the Alaska pipeline. It is easy to understand why oil defeated coal by 1950 as the lead energy source for the world.
Yet, despite many improvements from well-head to gasoline pump, distribution of oil is still clumsy. Fundamentally, oil is stored in a system of metal cans of all sizes. One of these cans was called the Exxon Valdez. Transfer between cans is imperfect. This brings out a fundamental point. The strongly preferred configuration for very dense spatial consumption of energy is a grid that can be fed and bled continuously at variable rates. There are two successful grids, namely, gas and electricity.
Natural gas is distributed through an inconspicuous, pervasive, and efficient system of pipes. Its capillaries reach right to the kitchen. It provides an excellent hierarchy of storage, remaining safe in geological formations until shortly before use. Natural gas can be easily and highly purified, permitting complete combustion.
Electricity, which must be made from primary energy sources such as coal and gas, is both a substitute for these (as in space heating) and a unique intermediary to a variety of devices that exist only because electricity became widely available (Starr, 1990). Electricity is an even cleaner energy carrier than gas and can be switched on and off with great effect. Electricity, however, continues to have the disadvantage that it cannot he stored efficiently, as today’s meager batteries show. It also loses in transmission; with present infrastructure, a span of 1000 km is about the economic limit. Lacking reserves in time and space, the electric power system is largely shaped by maximum rather than mean demand. Because mean demand is typically one-half of peak, an adequate electrical system looks large. It also looks inefficient to an engineer or banker, who wants expensive capital stock to be hard at work 24 hours per day rather than merely poised for action that may rarely come. Moreover, because of its limited storage, electricity is not much good for dispersed uses, such as cars.
The share of primary energy used to make electricity has grown steadily in all countries over the past 75 years and in the United States approaches 40 percent. In absolute terms, U. S. electricity growth, though irregular, has averaged about 3 percent per year the past 20 years. Nonelectric demand remains considerably larger. Because of inertia of capital stock and imperfect substitutability, the ratio will not now change rapidly. Thus, the core energygame for the next 30-40 years is to make a gas-electric system work as well as possible.
The No. 1 political task is to give a hand to natural gas and to reduce the biases in favor of other fuels. Legislative activity and subsidies have superannuated coal in the United States, Germany, Britain, Spain, Poland, and numerous other nations. Billions of public dollars per year prop up coal through fuel use requirements, pricing provisions, programs in fossil energy research and development, and government “cost-sharing” for clean coal plants. These subsidies should stop. Clean coal is an oxymoron, and the technologies that seek it are costly to install and complicated to maintain. Even as a source of electricity, coal is inferior. Though small, experimental plants may do better, coal-fired power stations typically achieve an efficiency of only about 30 percent. Gas turbine steam power plants are at 50 percent, and for this and other reasons can operate at costs equal to or below coal (Lee, 1989). They produce less CO2 and NOX, no ash, and none of the gypsum that comes from scrubbing coal. The United States should replace coal power stations with natural gas facilities and encourage this elsewhere.
We must tighten the noose around coal. Its use should be restricted to plants meeting stringent requirements for environmental performance. Globally, perhaps 50 to 100 billion tons more coal may be used (about 20 to 40 years of current consumption) before coal largely disappears from the market (Ausubel et al., 1988). If recent illness and accident rates are sustained, as many as 100,000 men will die directly from bringing it to market. It would be a good use of money to retrain miners for other work or to subsidize them to keep digging tunnels, but for purposes such as water supply, waste water, and transportation infrastructures.
If it is dusk for coal, it is mid-afternoon for oil. Oil has already been losing out in energy markets other than transport. The environmental challenges for oil are well understood, and pressure should be maintained to meet them. Over the next 10 to 20 years the United States should press worldwide for a tighter distribution system to address leaks, spills, and damaging forms of disposal; complete desulfurization of gasoline, heating oil, and diesel; and the elimination of lead and the associated use of catalytic converters and other techniques, including reformulated gasoline, to reduce CO and NOX. The continuation of oil usage can be tolerable for many decades with appropriate norms and controls.
For gas, the next decades should be a time of relative and absolute growth (Linden 1987, MacDonald, 1990). With estimates of the gas resource base having more than doubled over the past 20 years to very large volumes, we should encourage its adoption in the transport sector as well as for electric power (Burnett and Ban, 1989). There are already upwards of 700,000 natural gas vehicles in the world. Italy’s fleet leads the way, but numbers are growing from Brooklyn to New Zealand. Natural gas can do a great deal to clear the skies of Los Angeles, Denver, and Phoenix, not to mention Mexico City, Delhi, or Bangkok. It should be made easier to build and access gas pipelines.
Attention must, of course, be given to safety and environmental aspects of gas use. Pipelines and tanks can explode with tragic consequences. Natural gas is also a source of greenhouse gas emissions, though each unit of energy-produced oil on average yields about one-third more CO2 and coal two-thirds. Flaring and leakage of methane, itself a powerful greenhouse gas, should be reduced for environmental as well as economic reasons. Worldwide losses are probably about 2 percent of extraction but could and should be held below 0.5 percent.
So, energy history must not end with natural gas, and, in fact, it contains a simple, strong thread that suggests it will not: decarbonization. For 200 years the world has been progressively lightening its energy diet by favoring hydrogen atoms over carbon in our hydrocarbon stew (Marchetti, 1985). Coal has only one hydrogen for each carbon atom, oil two, and natural gas (methane), of course, is CH4. If we are concerned about greenhouse gas emissions, smog, and spills, decarbonization is the powerful, clear energy prescription. The global energy system has been moving in this direction but perhaps not fast enough, especially for those most anxious about climatic change. With business as usual, the decarbonization of the energy system will require another hundred years or so.
The success of decarbonization will ultimately depend on production and use of pure hydrogen fuel (H2) (Ausubel, 1990). Environmentally, hydrogen is the immaterial material; its combustion yields only water vapor beside energy. The hydrogen of course must come from splitting water–not cooking coal or another hydrocarbon source. The energy to make the hydrogen must also be carbonfree.
One of the advantages of shifting the energy system first to natural gas is that the hydrogen infrastructure will mimic and thus learn from that of gas. The transport technologies are basically similar. There are other steps that can be taken to hasten and deepen the penetration of hydrogen during the next decades (Dinga, 1989). Because hydrogen escapes and ignites easily, progress is needed on storage, especially at the smaller scale of end-use through such means as metal hydrides and light gas cylinders of fiber-reinforced resins with impervious liners. Leadership should come from the aerospace industry, which already uses liquid hydrogen as a rocket propellant. This is an industry that puts a great premium on safety precautions, leak detection, and lightweight strength and has access to capital and markets that allow it to use the best available techniques.
Naturally, one asks how the hydrogen will be made. Safe, efficient, cheap production of H2 should be one of the highest priorities of the government agencies for energy, transportation, and space as well as private industry. There are several alternatives, including solar and photovoltaic routes. I also believe this is the most promising niche for nuclear systems. I am just old (or young) enough to have been impressed by school books of the 1950s and 1960s that argued that splitting the atom was the greatest technological breakthrough since the harnessing of fire. We have been impatient. It seems quite reasonable that it will take 50 to 75 years to understand how to use atomic power. I agree, however, with critics of nuclear power that fission is a contrived way to boil water and extravagant if required only about half of each day to make electricity for our homes and businesses.
What seems special about nuclear energy is its potential as a source of electricity for electrolysis and high temperature process heat while the cities sleep. Nuclear electricity and heat could make H2 on the large scale needed to meet the demand of billions of consumers. If temperatures above approximately 800oC can be economically sustained, wider kinetic possibilities exist, and the job of making ample hydrogen through thermochemical reactions may be much eased.1 For this level of heat high temperature gascooled reactors (HTGRs) are an appealing line for development. Any design for a new generation of reactors must feature inherent safety, and HTGRs appear to do so (Brown, Boveri, and Cie, 1985). Excessive elevation of temperature stops the reactor. Fissionable material is in the form of particles less than 1 mm in diameter sealed in fuel elements the size of tennis balls, each of which provides its own containment and does not release radioactivity even if the reactor is abandoned. Reactors to produce hydrogen could be far from population concentrations and pipe their main product to consumers.
This quick survey of our evolving energy menu leaves open at least two important and related questions: What about efficiency and what about developing countries? With regard to efficiency, I take the engineer’s perspective that increasing efficiency is embedded into the lines of development of any machine or process. Measured in terms of fundamental performance, systems of transport, communications, farming, energy, and so forth, have been getting more efficient, with very few exceptions. In spite of market failures and other obstacles, efficiency improvements are well documented for everything from aircraft and autos to air conditioners and ammonia production (Nakicenovic and Grübler, 1989). Motors, light bulbs, refrigerators, and building materials will become more efficient, as will overall systems. An important opportunity may involve superconducting materials for transport and storage of electricity. What is new is not efficiency gains but their recognition.
The harder question may be lifestyles and behavior. We live in more numerous and smaller families. We want more square feet per capita in our residences. We want personal vehicles. We want to travel further and therefore at higher speed. We rarely reuse or repair products, though some products are becoming smaller and lighter. In short, the outlook for decarbonization is good, but the outlook for aggregate reductions in the materials (or embedded energy) that an individual consumes, that is, dematerialization, isuncertain (Herman et al., 1989).
A corollary concern is that developing countries, especially China and India, may rapidly recapitulate the developing pattern of the rich countries of the North on a larger and even more environmentally destructive scale. For example, it is feared that China will massively expand its coal use. I do not believe this need or will happen.
Twenty years of studies show that there is only one global energy system. Connected by common technology, capital, and information, it is dynamic, and all nations are coupled to it. The same year that Darwin published his theory of evolution, Colonel Edwin Drake drilled the first oil well in Titusville, Pennsylvania, and Chinamen soon lit kerosene lamps. Naturally, some nations are early adopters of technologies and others hop late onto the bandwagon. Thus, if one looks at the changing use of boat, train, bus, and plane for intercity transport in China, the evolution of market shares resembles the rest of the world, with perhaps a 30-year lag. However, the diffusion of technologies in nations or regions that are late to adopt is not nearly as pervasive as for the early adopters.
In fact, China would need to increase its rail network twenty times to achieve a spatial density comparable to that obtained by the industrialized countries in the 1920s when they relied most on coal (Grübler, 1990). China’s railroads, the infrastructure of the coal economy, are already declining. Instead, in China as elsewhere motor vehicles and aviation are ascending. The nineteenth century industrial paradigm is forever gone. Do not expect to find coal-fired buses or coal-fired 747s on a future visit to Beijing. The structure of end-use demand, including the density of population in China’s coastal plain, strongly favors natural gas and electricity.
Some argue that a viable alternative is for China to build many advanced coal plants near coal resources and a system to transport the electricity rather than the coal. But why bother with coal if there is gas? A major natural gas discovery in one of the poorest areas of China was announced in 1991 (The New York Times, 25 June). If the North wishes to lessen concern about environment and development, technologies for exploration and drilling for natural gas and loans for gas infrastructure should be top priorities for development cooperation. Recall that 90 percent of all hydrocarbon drilling has been in the United States and Canada, so that low estimates of reserves in many areas may reflect scant searching rather than dry earth.
At the same time, the ecological modernization of the North may have worrisome short-term effects on the poor countries of the South. Nature, especially carbon, is by far the largest export of the South and the former Soviet Union as well. The commodity price flares of the 1970s were followed by lasting reductions in imports to the North of products such as copper and major economic problems in numerous developing countries. If the North becomes vastly more efficient and frugal in resource use and reliant on indigenous energy, the developing countries may be pinched again. Of course, the potential energy frugality of the rich also provides an environmentally attractive pool of power to meet the demands of growing economic sectors and populations.
Overall, the trend of technological evolution evokes optimism about the relation of energy and environment. We are seeing the light. Scientists and engineers can do much to clarify the evolution underway and pave the way for favorable developments. The next decades are the time to realize the vision of the hydrogen economy first outlined twenty years ago (Gregory, 1973).
The environmental danger remains more in the potential than in what has so far happened. In fact, only a few percent of the Earth’s surface are needed for human settlements. Though much of the surface has been transformed by human action, less than one percent of the land is covered by human artifacts. Fifty five percent of the United States’s population concentrate on 10 percent of its area. The Earth’s woods still cover 80 percent of the territory that they covered 3,000 years ago. In Europe the stock of forests may have grown 25 percent between 1971-1990 (Kauppi et al., 1992). The amount of land used for farms globally has been unchanged for 40 years. Genetic engineering and dietary changes could further free land from food production as tractors liberated peasants from stoop labor. We can choose to leave much more of the land unturned as well as avert massive alteration of the composition of the atmosphere.
Though hydrogen in principle can cleanly power 10 billion people in a hundred megacities, the energy system depends on long-term social acceptance and, therefore, must be seen as part of a larger environmental solution that is cultural and technological. For some time, it has been evident that modern societies are well organized to research and develop specific actions to correct problems. To be effective, such actions must mesh with attitudes and behaviors, for example, about what is considered harmful and how time is allocated. A Great Renunciation of economic life and material goods does not seem near nor in the interest of many. Yet, some renewed concept of “peace with nature” is surely required in which to embed our engineering design and to set totems and taboos for production and consumption (Meyer-Abich, 1986).
In animism ancient religions incorporated powerful brakes to the destructive actions, which the technical means always available to humanity permit. At the siege of Massilia (modern day Marseilles), Julius Caesar was short of wood and ordered his soldiers to fell a sacred grove. The soldiers trembled at the idea of wounding the annointed oaks and refused. Angered, Caesar took an axe and chopped down a tree. Perhaps unfortunately for Western civilization he went on to triumph in a series of battles, in fact, killing or enslaving half the able-bodied men of Gaul and devastating its towns and agriculture (Holmes, 1911).
It is progress that George Washington, the father of his country, was filled with regret when he cut the cherry tree. Alas, his response stemmed from characteristically pragmatic American considerations, not from feelings for natural objects. We must check our overenthusiastic cutting and burning and more generally our arrogant view of nature in favor of a more democratic view of all creation (White, 1968). We must recognize that multiplication and materialization jeopardize our own success in the Darwinian struggle for life and that such success must be redefined. Markets, among the most sophisticated systems of information and feedback, can do much to foster and channel the evolution of energy systems. To balance the myopia of markets, ethical and aesthetic criteria must specify the energy system as well as budgets, costs, and economies of scale.
To review, I believe the leading influence on the national and world energy diet will be the daily routines of the great population concentrations. The cities must be fueled in a safe, healthy, and environmentally attractive way for their own sake and to preserve the rest of the planetary surface. The United States and other industrialized nations must favor natural gas more strongly, both domestically and elsewhere. Meanwhile, we must firmly endorse decarbonization, take further actions to keep it on course, and prepare the way for hydrogen. We must take care that the ecological modernization of the North does not worsen the position of the South. We should seek to encourage in our culture dematerialization and the reanimation of Nature.
End Note
1 Present thermochemical cycles require temperatures as high as 1800oC, but an aggressive search holds promise of success at lower temperatures in a decade or two.
References
Ausubel, J. H. 1990. Hydrogen and the green wave. Bridge 20(1):17-22.
Ausubel, J. H., A. Grübler, and N. Nakicenovic. 1988. Carbon dioxide emissions in a methane economy. Climatic Change 12:245-263.
Brown, Boveri, and Cie. 1985. Nuclear power stations with high-temperature reactors. DHRB 1023 85 E. Mannheim, Germany: BBC Brown Boveri.
Burnett, W. M. and S. D. Ban. 1989. Changing prospects for natural gas in the United States. Science 244:305-310.
Dinga, G. P. 1989. Hydrogen: The ultimate fuel and energy carrier. International Journal of Hydrogen Energy14:777-784.
Gregory, D. P. 1973. A hydrogen-energy system. L21173. Washington, DC: American Gas Association.
Grübler, A. 1990. The rise and fall of infrastructures. Heidelberg: Physica.
Herman, R., S. A. Ardekani, and J. H. Ausubel. 1989. Dematerialization. In Technology and environment, eds. J. H. Ausubel and H. E. Sladovich, pp. 50-69. Washington, DC: National Academy.
Holmes, T. R. 1911. Caesar’s conquest of Gaul. London: Oxford University.
Kauppi, P. E., K. Mielikainen, and K. Kuusela. 1992. Biomass and carbon budget of European forests, 1971 to 1990. Science 256:70-74.
Lee, T. H. 1989. Advanced fossil fuel systems and beyond. In Technology and environment, eds. J. H. Ausubel and H. E. Sladovich, pp. 114-136. Washington, DC: National Academy.
Linden, H. R. 1987. Learning from past mistakes in energy policy. Bridge 17(3):15-21.
MacDonald, G. J. F. 1990. The Future of methane as an energy resource. Annual Review of Energy 15:53-83.
Marchetti, C. 1975. Transport and storage of energy: RR-75-038. Laxenburg, Austria: International Institute for Applied Systems Analysis.
Marchetti, C. 1985. Nuclear plants and nuclear niches. Nuclear Science and Engineering 90:521-526.
Meyer-Abich, K. M. 1986. Peace with nature, or plants as indicators to the loss of humanity. Experientia 42:115-120.
Nakicenovic, N. and A. Grübler. 1989. Technological progress, structural change, and efficient energy use: Trends worldwide and in Austria: International part. Laxenburg, Austria: International Institute for Applied Systems Analysis.
The New York Times, 25 June 1991, Section D, p. 9.
Starr, C. 1990. Implications of continuing electrification. In Energy: Production, consumption, and consequences. ed. J. L. Helm, pp. 52-74. Washington, DC: National Academy.
White, L. Jr. 1968. The historical roots of our ecologic crisis. In Machina Ex Deo: Essays in the dynamism of Western culture, pp.75-94. Cambridge: MIT.