Citation: World Energy Council Journal July: 8–16 1998
Keywords: natural resources, land use, population, gdp, energy
Areas of Research: Technology & Human Environment
1. Introduction
The marathon of economic development kicks up clouds of questions about resource and environmental stresses. In the end, they come down to two. Will there be enough resources to serve humanity? Will the environmental fallout from the use of resources harm other life and us?
The expected answer to each question is “yes.”
As I will report, my search of the book of history and my projections for the 21st century based on the historical patterns I find suggest that many of the usual specters of shortage and fallout are phantoms. Instead, I see a society learning to use resources efficiently and cleanly. Keys described along the way include market substitution, precision agriculture, dematerialization, decarbonization, and industrial ecology. Unfortunately, I will not leave you, the reader, without worries. But, I hope to shift attention from the spell-binding phantoms to real stresses that we should sweat to relieve. Let us not exhaust ourselves chasing phantoms, when the race before us is anyway long and hard.
First, I offer a few words to describe the two multipliers of resource and environmental factors: Population and GDP per capita. As I proceed, the divisors will also emerge.
Population: Humans now number about 5.8 billion. Annual growth peaked near 2% in 1970 and has dropped below 1.4%. More than 90% of growth is in the so-called Developing Countries. In fact, the populations of one set of countries are exploding while others are imploding. Combinations of fertility rates near or more than the 2.1 children per woman that replaces present population plus increases in length of life make explosions in countries such as India, Iran, and Nigeria. Fertility far below replacement and populations already near the present limit of longevity start slow implosions in countries such as Japan and Italy. For example, if Japanese women continue bearing about 1.4 children, the population of Japan will fall from about 125 million today to 55 in the year 2100. US population seems likely to grow about 50% over this period, from about 270 million to about 400. Most demographers expect world population to reach 10 billion by 2100, a compound growth rate from today of about 0.5%/yr. However, because no one knows how many children parents will choose in the future, this number may be a way station upward, a plateau, or a peak before a fall.
GDP per capita: Over long periods, including the booms and busts no economy has learned to escape, GDP per capita grows slowly. For example, the annual rise of GDP per person in the US has averaged 1.7% since 1900 and 1.4% since 1985. Between 1965-1996 the GDP per Mexican rose 1.7% yearly, per Indian 2.4%, and per Japanese 3.7%. The present slump in Asia reminds us that economies rarely sustain high growth for more than two to three decades.
Combining annual growth of population and GDP per population gives GDP growth, the total multiplier of resource and environmental matters. Since the start of the industrial revolution, GDP in the US and UK has risen about 2.3%/yr. During the long run ahead, global growth of between 2% and 3%/yr again appears likely. Unmodified by a divisor, compounding by 2.3% doubles a resource demand or an environmental impact in about 30 years, quadruples it in 60, and multiplies it eightfold in about a century. By this arithmetic, world GDP itself would rise from about $25 to $200 trillion, giving 10 billion people $20,000 each in 2100.
2. Will there be enough?
Energy: Globally over the past two centuries, a succession of primary sources has held the largest share of the energy market: first wood and hay (dominating until the 1870s), then coal (peaking in the 1920s), and oil (peaking in the 1970s). If the succession continues, natural gas will move ahead, peaking around 2030.
So far, humanity has burned about 180 billion tons coal equivalent (btce), a reference point for the other fuels. We have burned about 75% as much oil (130 btce), and 40% as much natural gas (75 btce). In a scenario of continuing market substitution, perhaps 100 btce more of coal will be used, 300 btce of oil, and 1000 btce or more of gas. This scenario implies a worldwide phase-out of coal during the next 50 years at 2.5%/yr, leaving huge reserves underground.
Proved oil reserves (which exceed 200 btce) and resources can meet the oil demand. Though the bulk of its use lies ahead, oil will fade from prominence. Beginning in a decade or so, fuel cells drawing on natural gas and, later, neat hydrogen will power the world’s vehicles. The mean secular decline in oil production may be 1.5%/yr.
Although the upper bound of estimates of technically recoverable natural gas resources is now about 700 btce, evidence is growing of widespread and more abundant methane than traditional geological theory holds. This evidence lifts estimates of gas steeply even in the US and keeps prices low. I match this optimism with a caution and a business opportunity. Expanding demand for gas, at perhaps 4%/yr, will require construction and operation of pipelines and other means for shipment with much larger and more extensive capacity than now.
Globally, more than one-third of primary fuels now becomes electricity, twice the share 50 years ago. Still, two billion people lack electricity. The unique ability to run information machines plus opportunities in transport now powered by oil will combine with the billions yet to be wired to grow electric demand. Demand may conceivably grow from the present 1.5 terawatt (TW or 109 kilowatt) years to 30 TW yrs or more in 2100. Ten billion people using today’s US electricity per capita would consume about 15 TW yrs.
Dividing the multipliers of population and GDP, efficiency in generation, transmission, distribution, and end-use will temper demand. Efficient gas turbines appear the generator of choice for a broad mid-range of additions to capacity. At prices up to about $4.50 per million BTU, twice the recent average price, gas should gradually drive coal from power stations. Approaching a $200 trillion economy, annual gas use could peak at 30 x 1012 m3, an order of magnitude above oil’s peak use. Absolute gas use should peak about the year 2060.
Notwithstanding hopes, the so-called solar and renewable energies (wind, photovoltaics, biomass, et al.) still show promise only for small niches. Solar enthusiasts tend to stress that solar energy is free. All natural resources are in fact free. What costs is mobilizing the resources and making the products flow to the consumer in the proper form and amount. Here the solar and renewable proposals continue to bog down. Even with technical breakthroughs, these sources, and their hard counterpart, fusion, would take a long, long time to reach a consequential level.
Making gigawatts at each site, nuclear plants already add consequential amounts of energy to the world’s energy supply and continue to offer advantages for large additions to capacity. Either once-through or recycled uranium can fill likely nuclear demand. A scenario of high demand invokes worries about uranium supplies and thus the recycling, which so-called breeder reactors can perform. While we are sheltered by the main reliance of the energy system on natural gas for the next 60-70 years, the growth of nuclear energy has time to resume. Generating large amounts of both electricity and hydrogen in the latter part of the 21st century, nuclear should become the primary source of energy.
Continuing substitutions of one source for another should amply supply 21st century energy markets. The rapid growth of gas supply, the gradual penetration of nuclear energy, and the inexorable drive toward efficiency relieve the stress.
Land and Food: For centuries, farmers expanded cropland faster than population grew, and thus cropland per person rose. Fifty years ago, farmers stopped plowing up more nature per capita (Figure 1). Meanwhile, growth in calories in the world’s food supply has continued to outpace population, especially in poor countries. Per hectare, farmers lifted world grain yields 2.1 percent annually between 1960-1996.
Frontiers for even more agricultural improvement remain open. On the same area, the average world farmer grows only about 20% of the corn or beans of the top Iowa farmer, and the average Iowa farmer lags more than 30 years behind the yields of his most productive neighbor. Top producers now grow more than 20 tons of corn per hectare compared with a world average for all crops of about 2.
Globally, the future lies with precision agriculture. Technology and information help the grower use precise amounts of inputs-fertilizer, pesticides, seed, water-exactly where they are needed. Precision agriculture includes grid soil sampling, field mapping, variable rate application, and yield monitoring-tied to global positioning.
If during the next 60 to 70 years, the world farmer reaches the average yield of today’s US corn grower, ten billion people will need only half of today’s cropland while they eat today’s US calories. The land spared exceeds the Amazonia. This sparing will happen if farmers maintain the yearly 2.1% worldwide growth of grains achieved 1960-1996. In other words, if innovation and diffusion continue as usual, feeding people will not stress resources. Even if the rate of improvement falls to half, an area the size of India, globally, will revert from agriculture to woodland or other uses. A vegetarian diet of 3,000 calories/day halves the difficulty or doubles the land spared.
Success at growing food on land spares animals in the seas, where fishers stress many wild stocks. So can farming parts of the ocean. Just as fertilizer grows more corn, adding nutrients to the barren tropical ocean might, for instance, multiply selected fish a thousand times.
Despite more calories on average, a billion people remain hungry. Their number could grow or shrink, depending above all on distribution of income. Meanwhile, the continuing evolution of efficient use can assure ample resources on average.
Forests: Forests are cut to clear land for farms and settlements and also for fuel, lumber, and pulp. In the rich countries, nevertheless, forests have re-grown in recent decades. Since 1950 the volume of wood on American timberland has grown 30%, while European forests have similarly increased in volume and extent. In the US, the intensity of use of wood defined as the wood product consumed per dollar of GDP has declined about 2.5% annually since 1900. In 1993 an average American consumed half the timber for all uses as a counterpart in 1900.
In the US, likely continuing fall in intensity of use of forest products should more than counter the effects of growing population and affluence, leading to an average annual decline of perhaps 0.5% in the amount of timber harvested for products. A conservative 1.0% annual improvement in forest growth would compound the benefits of steady or falling demand and could shrink the area affected by logging 1.5% annually. Compounded, the 1.5% would shrink the extent of logging by half in 50 years. If one half of this amount occurs by leaving areas now cut uncut, the area spared is 50 million hectares, the size of Spain. A similar pattern is likely in Europe as well as Argentina and numerous other countries.
In poor regions of tropical countries such as Brazil, Indonesia, and Zaire, the dominant force stressing forests remains the struggle to subsist. During the last couple of decades, the removal of tropical forests has been estimated at 1 percent per year. Until overcome by better livelihoods, cheap land, cheaper fuels, superior alternative wood products in the marketplace, taboos, or other factors, the one-time conversion of forests to money, cropland or fuel will continue. Nevertheless, global expansion of forests and rising incomes encourage.
Water: Globally abundant, fresh water is unevenly distributed. Although ten times present use falls on Earth, only about 1/5 of this is easily reached or used. Almost 2/3 of water goes for farming, 1/4 for industry, and less than 1/10th for cities. Hydroelectric generation currently uses (but does not consume) 2.6 times the average runoff in the conterminous US by running water through several turbines in a river. In the US both total and industrial withdrawals appear to have peaked in the 1970s (Figure 2).
Opportunities to increase efficiency abound. Crops take up only about one-third of what is applied. Shrinking the area of farmland would likely save much water, even if the fraction irrigated rises. Industries and cities can recycle wastewater, if energy is cheap and pollutant removal does not cost dear. History shows that when water becomes scarce, use becomes efficient.
The time to design and build water infrastructures stretches for decades. International and continental management remain novel. Climatic variability and change make the diversity of wide nets of water infrastructures and markets attractive, but they may be hard to arrange. Because a major cost is pumping, the key of cheap energy can relieve stresses.
Materials: During the past two decades metals, industrial minerals, and construction minerals as well as energy, forest, and agricultural products have not grown more costly despite the rise of the multipliers of population and GDP. An average American, for example, consumes about 50 kg per day of these materials. Fuel forms about 40% of the material inputs and most emissions (Figure 3). Although the mix differs, wealthy countries such as Japan, the Netherlands, and the US consume about equal kilos.
Because suppliers, such as miners, have vastly improved their efficiency, iron, copper, and other ore previously considered low grade still yield products and profits. Meanwhile, efficiency for the consumer in product design and changes in consumption patterns have favored dematerialization, less material per dollar of GDP to do a task. Lowering the material intensity of the economy could preserve landscape and natural resources, lessen garbage, and reduce hazardous exposures. Economical energy, efficiency, and clever upgrading of plentiful materials divide the demand that population and GDP multiply and make sustained or widespread shortages of materials unlikely.
3. Will the fallout harm?
Habitat: People transform land by building, logging, and farming. Globally, if an additional 4 billion people pave and otherwise develop land at the present rate of Californians (about 600 m2 each), cities will consume about 240 million hectares, midway in size between Mexico and Argentina. This area appears likely to be offset by land spared from logging in the US and other countries that now reduce their cutting of forests. The likely added land spared from crops globally over the time it takes to reach 10 billion people suggests a net worldwide return to Nature of lands equal to India (3 Nigerias or 6 Spains). The distribution of these lands will greatly affect the chances recreated for habitat for flora and fauna.
Climate: The 30% increase in greenhouse gas concentrations, particularly carbon dioxide (CO2), since the start of the industrial revolution may already have changed climate some, and more seems likely. How much depends on the rate of decarbonization of the energy system. Decarbonization means we obtain more energy and income per carbon atom. The evolving shares of the leading primary energy sources (wood & hay, coal, oil, gas) mix hydrogen and carbon in increasing ratio, and have in fact caused a gradual, global decarbonization of the energy system (Figure 4). Sources with no carbon at all, such as hydro, nuclear, and solar decarbonize further.
Now, additions to atmospheric carbon dioxide come mostly from transport (oil) and power generation (coal), but they will come largely from gas when it dominates energy supply. Fuel cells, essentially continuous batteries fed by hydrogen extracted from natural gas or manufactured by nuclear plants, and other electromotive propulsion, promise to multiply automotive efficiencies soon. Fuel cells can produce zero pollutants and correspondingly reduce carbon emissions from transport.
To stabilize atmospheric CO2 at twice pre-industrial levels (550 ppm) probably requires limiting emissions from 1990 onward to about 900 billion tons of carbon. My decarbonization scenario emits about 500 billion tons and could stabilize atmospheric concentrations about 450 ppm, one-quarter above present levels. Still lower levels could be achieved by sequestering carbon, for example, in aquifers whose primary rocks are silicates that form stable carbonates plus silica from CO2.
The consequences of climate change depend on the adaptivity of plants, animals, and social systems. Analysts have produced fragile and robust scenarios and everything in between. In general, the adaptable and richer are safer.
Acidity: The precipitation on forests and lakes of acid-producing residues, mainly in the form of industrially released sulfur dioxide (SO2) and nitrogen oxides (NOx), emerged in the 1970s as a major issue in North America and Europe, and to a lesser extent in East Asia. In the US, SO2 emissions come primarily from electric power plants and have dropped more than a third since 1970. NOx emissions, from automobiles as well as power plants, peaked about 1980 and have fluctuated since. Gas turbine power plants now replacing plants built before the 1972 Clean Air Act can drop NOx by 95%. Curiously, NO3 concentrations have not changed in several decades in the rain at the carefully monitored Hubbard Brook (New Hampshire, US). Decreased emissions of SO2 are evident in routine measurements of rainwater sulfates, but rainwater remains more acidic in the affected regions in the US than before widespread industrialization. Red spruce trees, among the vegetation apparently most susceptible to acid rain, show diminished growth, although the case for an acid cause remains weak.
Acid from afar is also deposited in Japan from Chinese and Korean emissions, but long-term records of the extent do not exist. Although emission, transport, and deposition of acid-causing emissions must occur elsewhere, especially where fossil fuels are heavily used, sparse data and knowledge of regional weather cloud assessment. The numerous other natural and anthropogenic changes pressing upon ecosystems make hard the attribution of effects to acid rain. In regions such as southern Poland, the Czech Republic, and eastern Germany, stresses may have been severe, but coal use and raw industrial activity have dropped drastically. For the next few decades, coal-using regions such as China will be prone, but over the long run shifts to clean-burning natural gas and cars powered by fuel cells will largely eliminate concern about acid deposition.
Increased ultraviolet: Depletion of stratospheric ozone, traceable to chemicals used primarily for refrigeration, but also fire retardation, aerosol propulsion, and cleaning could increase ultraviolet light. The increase in turn could harm people and affect the productivity of ocean plankton and land plants. Production and use of the main culprits, the chlorofluorocarbons (CFCs), concentrated in the industrialized countries. Production grew steadily in the early 1970s and leveled later in the decade, when the US and a few other industrial countries banned particular uses of CFCs.
International protocols on substances that deplete the ozone layer, signed in 1987 and amended in 1990 and 1992, phased out fifteen CFCs by 1996. By 1993 industries completed phase out of halons, another ozone-depleting substance. Developing countries have a 10-year delay in implementation. The detection in the mid-1980s of a “hole” in the ozone layer in the spring over Antarctica catalyzed signature of agreements. Evidence that less ozone caused more ultraviolet radiation at the surface of Earth remains elusive. The decisions curtailing production of the suspect chemicals appear to be causing anthropogenic ozone destruction to peak around now, with recovery in prospect over a few decades.
Urban and indoor air: London invented smog, but Los Angeles (LA) brought it fame and remains the benchmark of urban air in industrialized nations. In 1997, thanks to cleaner cars and fuels, and tougher regulations for power plants and refineries, the air in LA was the cleanest in 50 years. In 1997 LA issued only one smog alert, and air in its basin exceeded federal limits on ozone in the lower atmosphere on 68 days, down from 90 in 1996. The main reason is new cars that emit 95% less than 1970 vehicles. The near elimination of leaded gasoline in the US explains division of airborne lead by 20. Fine particles emitted by gasoline and diesel engines are, however, correlated with increased mortality.
In developing countries, large cities suffer acute and unabating air pollution. During the 1980s, the Chinese cities of Beijing and Shanghai exceeded World Health Organization (WHO) standards for particulate levels on 272 and 133 days per year. The average in New Delhi over the same period was 295 days. Since the mid-1970s, SO2 levels exceeded the standard an average of 100 days per year in Teheran. In 1991 in Mexico City standards were violated over 300 days. Wood and dung burning in Asian ovens raise indoor particulate concentrations more than one hundred times the WHO standards, illustrating indoor air pollution. Over the long run, natural gas and nuclear energy, that is, decarbonization, will largely eliminate urban air problems.
Waters: Groundwaters, many rivers in both developing and industrialized regions, and inland water bodies, such as the Aral Sea in Central Asia, continue to experience contamination from both active and inactive industrial sites, imprudent irrigation, and diffuse pollution from urban, fertilizer and pesticide runoff. Some estuaries and seas now manifest incipient eutrophication or hypoxia: dissolved oxygen reduced by more nutrients, such as those found in fertilizers and sewage. The growth of some plants and the loss of oxygen choke off other aquatic life. Shrinking cropland is likely to lower use of nitrogen and other fertilizers. In fact, fertilizer use has been flat in the US since 1980 and has dropped 10% globally since the late 1980s.
Some waters have been reclaimed. For example, although much remains to be done, the average dissolved oxygen in the rivers of the OECD nations improved over the past twenty-five years. Stresses, however, will worsen along coastlines, where urbanization proceeds faster than improvement of wastewater infrastructure.
Since 1970 oil spills have fluctuated with sporadic spikes, as due to the Exxon Valdez in Prince William Sound in 1991. Tanker accidents were fewer in the 1980s and 1990s than the 1970s. Improved technical standards for transport over the last few decades probably caused the improvement. Although commanding less public attention than spills, “normal” discharges of oil into the sea from washing tanks and discharging ballast water form the largest source of marine oil pollution and remain hard to assess. Shifts to natural gas and hydrogen would obviate oil spills as well as oil runoff from roads on land.
Industrial and municipal wastes: Population and GDP growth multiply the volume of products and objects. Thus, total wastes have tended upward despite their decline per GDP. The sophisticated materials and their applications valued in the “new materials society” make alloys and composites that can be hard to separate and recycle. For many materials, recycling seems unable to supply more than about 1/3 of demand. Popular materials can be lighter but bulkier and more toxic. So far, higher disposal prices have called forth sites for landfills, but who shall live near the dump always stresses. The vision of an industrial ecology in which waste is radically reduced and emissions approach zero is beginning to be realized in cities such as Kalundborg, Denmark, where plants are co-located so that the “wastes” from one become useful inputs to another.
Radioactive and other hazardous materials: The storage and disposal of radioactive wastes have implications from global to local. They come from nuclear reactors making electricity and arsenals making bombs. The public worries about low as well as high level radioactive wastes. Although high level volumes are smaller, they present harder problems.
The rise of nuclear electrification has raised the volume of spent fuel and other wastes, but it is still small. The waste volumes, in fact, are smaller than anticipated. In the US, for example, the current dumps for low-level radioactive waste will have excess capacity well into the next century, partly because fewer plants were built than projected, but mostly because recycling and compacting have shrunken the waste volume. In the US the annual volume of low-level waste shipped to disposal sites dropped from 3.8 million cubic feet in 1980 to 422,000 in 1996. The decline means that the three dump sites in Richland (Washington), Barnwell (South Carolina), and Clive (Utah) have 29 to 260 years of capacity at current disposal rates, which will probably drop even lower.
Wastes from weapons contribute much to total nuclear waste. In the US, the environmental problems of weapons operations are widely known, and prompted large appropriations to remedy them. In the former Soviet Union, the problems may be greater. Formal treaties have stopped earlier disposal, such as dumping at sea, and the scuttling of nuclear submarines has ceased. Regimes for transport and temporary storage of civil and defense nuclear wastes now function, although sites and designs for permanent disposal have yet to be reliably accepted.
The prevalence of several other hazardous materials has diminished in recent decades. Strontium-90 has diminished worldwide since the 1960s when atmospheric testing of nuclear weapons was banned. In the United States, levels of PCBs (used as coolants in power transformers) and lead (used in various forms in gasoline, cables, pipes, paint, and industrial chemical processes) and other heavy metals have declined dramatically as harmful consequences have been identified and remedies implemented. Despite bans, the persistence of the durable PCBs has kept them a leading topic of toxicological research and environmental controversy. Previous disposal of these and other hazardous wastes has contaminated many locations around the world, and the catalogue of these sites has grown. In the United States, while documentation and remediation of previously contaminated sites goes forward, few new sites are polluted.
I have searched history and found many of the specters of shortage and fallout are phantoms. Unfortunately, I must tell you that some other stresses are real.
4. Where are stresses likely?
In cities: In August 1997 the Chinese Ministry of Labor estimated that about 175 million surplus laborers linger in the countryside, representing 35% unemployment. Continuing rapid urbanization in China, parts of Africa, India, and other areas retaining large rural populations will press on water supply, waste treatment, energy, housing, and other infrastructures. Crowded, unsanitary, and unsafe conditions will bring deadly catastrophes, including epidemics, fires, and building collapses, already familiar in the overstuffed and underserved cities of today.
At cultural borders: Wealthy imploding regions such as Europe will draw into their empty rooms migrants from the poorer exploding regions such as the Mahgreb. Few countries have maintained peace when national and cultural boundaries do not coincide. Africa today is probably worse off in this regard than Europe in 1913.
Where systems leak: Best practice in industrial ecology offers zero emissions. In the world’s $200 trillion economy, however, worst practice will surely still lag behind best practice, some sectors will evolve along bad tracks, and small errors can be absolutely huge. Normal as well as surprising failures in plant operations and product design, corruption, stupidity, and carelessness will continue. Plants will leak, pipelines and tanks will explode, cement will crack, and water, air, and soil will be contaminated.
In scaling up: Within fifty years more than 3 billion people need to hook to commercial energy for the first time. China and India need more than their current 1 phone line per hundred tongues. Without capital, domestic tranquility, zero-maintenance technologies, and new economies of scale, stresses will run high, mistakes will be made and need correction, and transitions to new ways will test patience. The question of who is the next to gain service always arises.
In critical infrastructures: We move ever further from the society in which a family could farm, fuel, and clothe itself. No family can make microprocessors or storage disks. A winter ice storm shows how developed societies increasingly depend on a few, linked infrastructures. In particular, because electricity permeates the web of social services, a breakdown, even for a few hours, can bring tragedy. Neither can Internet commerce flourish, if the system is often down. To survive supply cartels, market fluctuations, war, and terrorism the wide natural gas distribution network that I envision must be an engineering masterpiece. In general, designing, building, and operating ultra-reliable infrastructures for electricity, gas, information, water, and other critical goods loom large.
For energy, the diffusion among individual consumers of multipurpose minigenerators might defend against great breakdowns. In effect, we would delegate base load to the global gas and nuclear system while leaving peaking and standby to a new household appliance able to use both gas and electricity to produce heat, cold, and electricity on demand.
In the innovation system: Although advancing productivity 2%/yr in key sectors may not sound hard, maintaining the pace for a century or more has exhausted earlier societies and enterprises, as the costliness of complexity tends to grow. Even if the world can maintain the supply of innovation, many societies historically have chosen to block diffusion. The loudest sound in the world is that of a habit breaking. Societies may choose dirt and disease, because accepting the means to lessen environmental and resource stresses will break the old culture, the tested formula for survival.
5. Conclusions
Will there be enough? There should be, though products in demand such as natural gas will surely give market power to prepared suppliers. And those with low incomes will always tend to be weak in the marketplace, though if the poorest multiply their income eightfold over today, most should be safe from hunger.
Will the fallout harm? Cities will spread and the climate may warm a little, but on balance humans may tread more lightly in nature.At the outset, we recognized the general multipliers, population and GDP. To answer the two basic questions, we now see we must address a third, “Must resource and environmental stresses intensify in unmodified lockstep with these general multipliers?” History suggests technology and science, which lift productivity and efficiency of resource use, are powerful enough divisors to lessen net stresses.
As well as the raising the divisors, people could choose to lessen the multipliers. However, habit favors multiplication, and so for the 21st century we should at least prepare for it.
A world of 10 billion people and $200 trillion will above all handle huge amounts of information, whether in its fields, factories, offices, or homes. Its greatest vulnerabilities may come from failures or rejections of the systems of control for the communication of information. Science is a uniquely effective system of communication for the control of complexity, and the ability to control complexity is effectively the central parameter of the dynamics of evolution. Having come far down the road with science and technology, perhaps we should feel greatest stress from knowing we must keep running.
Acknowledgements: I am grateful for many years of cooperative work with Arnulf Gruebler, Cesare Marchetti, Perrin Meyer, Nebojsa Nakicenovic, and Paul Waggoner and to Kurt Yeager and Chauncey Starr for asking the questions eliciting this paper.
Further reading:
The Environment for Future Business
Jesse H. Ausubel
Pollution Prevention Review 8(1):39-52, Winter 1998.
Technological Trajectories and the Human Environment
Jesse H. Ausubel and H. Dale Langford, eds.
National Academy, Washington DC, 1997.
Also appeared as special issue, “The Liberation of the Environment,” Daedalus 125(3), Summer 1996.
Elektron: Electrical Systems in Retrospect and Prospect
Jesse H. Ausubel and Cesare Marchetti, Daedalus 125(3):139-169, Summer 1996.
Toward Green Mobility: The Evolution of Transport
Jesse H. Ausubel, Cesare Marchetti, and Perrin S. Meyer
European Review 6(2):143-162, 1998.
The Environment Since 1970
Jesse H. Ausubel, David G. Victor, Iddo K. Wernick
Consequences: The Nature and Implications of Environmental Change 1(3):2-15, 1995.
Human Population Dynamics Revisited with the Logistic Model: How Much Can Be Modeled and Predicted?
Cesare Marchetti, Perrin S. Meyer, and Jesse H. Ausubel
Technological Forecasting and Social Change 52:1-30, 1996.
Lightening the Tread of Population on the Land: American Examples
Paul E. Waggoner, Jesse H. Ausubel, Iddo K. Wernick
Population and Development Review 22(3):531-545, 1996.
Searching for Leverage to Conserve Forests: The Industrial Ecology of Wood Products in the U.S.
Iddo K. Wernick, Paul E. Waggoner, and Jesse H. Ausubel
Journal of Industrial Ecology 1(3):125-145, 1997.
Jesse H. Ausubel is Director of the Program for the Human Environment at the Rockefeller University in New York City. Mr. Ausubel’s interests include environmental science and technology, industrial evolution, and the nature of the scientific enterprise. The main themes of the Rockefeller research program are industrial ecology (the study of the network of all industrial processes as they may interact with each other and live off each other, a field Mr. Ausubel helped originate) and the long-term interactions of technology and the environment.
Underlying the work are ongoing studies of the mathematics of growth and diffusion. From 1983-1988 Mr. Ausubel served as Director of Programs for the US National Academy of Engineering.
Figures
Figure 1. Land to Feed a Person for a Year, Global Average.
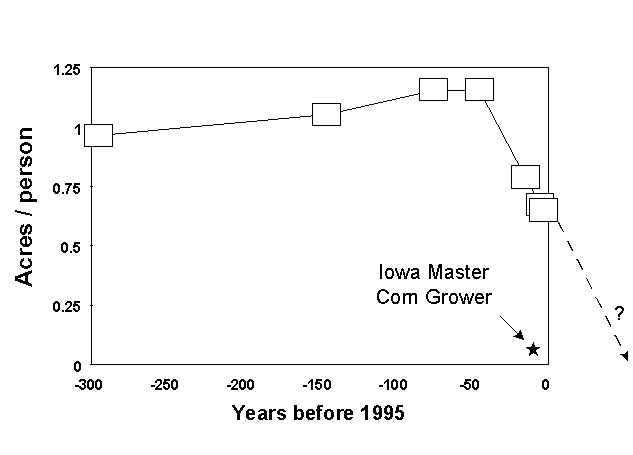
The blocks in Figure 2 show that global cropland per person expanded before Malthus until the mid-1950’s. Recently, however, cropland per person shrank. The star shows the land per person that would be required if global yields equaled the yield achieved by the winner of the Iowa Master Corn Growers Contest in 1996, 19.5 tons per ha.
Source of Figure: P.E. Waggoner, “Food, Feed, and Land,” in Ethics of Consumption: the Good Life, Justice, and Global Stewardship, D.A. Crocker and T. Linden, eds., Rowmand & Littlefield, Maryland, 1998. Sources of Data: J.F. Richards, “Land Transformation,” in The Earth as Transformed by Human Action, B.L. Turner et al., eds., Cambridge University, Cambridge, England, 1990; Food and Agriculture Organization of the United Nations, FAO Yearbook, vol. 45, FAO, Rome, tables 1 and 3; Rod Swoboda, “New Record Set in State Corn Contest,” Wallace’s Farmer, March 1997.
Figure 2. U.S. Total Water Use, Per Capita Per Day.
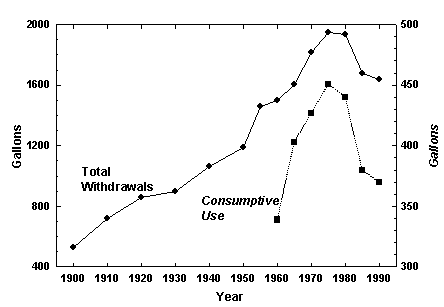
Source of figure: J. Ausubel, “Can Technology Spare the Earth,” American Scientist 84:166-178 (March-April 1996).
Sources of data: U.S. Bureau of the Census, Historical Statistics of the United States, Colonial Times to 1970, U.S. Government Printing Office, Washington D.C., 1975; U.S. Bureau of the Census, Statistical Abstract of the United States: 1992, 112th edition, U.S. Government Printing Office, Washington D.C., 1993.
Figure 3. Daily U.S. Per Capita Material Flows by Mass, Circa 1990.
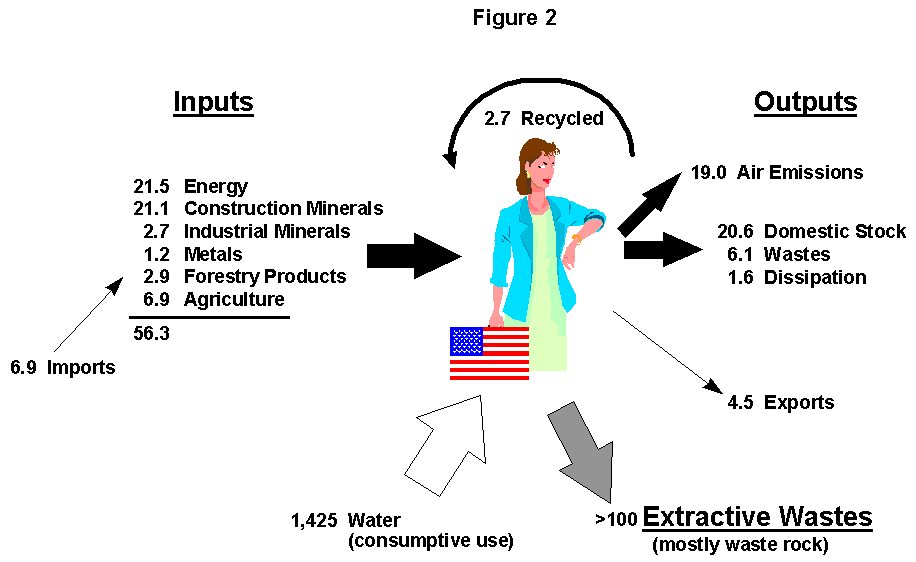
All values are in kg’s. Materials here are classed as energy fuels (i.e., coal, oil, gas), construction minerals, industrial minerals, metals, forestry products, and agricultural products.
Source of figure: I.K. Wernick, “Consuming Materials: The American Way,” Technological Forecasting and Social Change 53:111-122, 1996.
Source of data: I.K. Wernick and J.H. Ausubel, “National Materials Flows and the Environment,” Annual Review of Energy and Environment 20:462-492, 1995.
Figure 4. Decarbonization or World Ascent of Hydrogen.
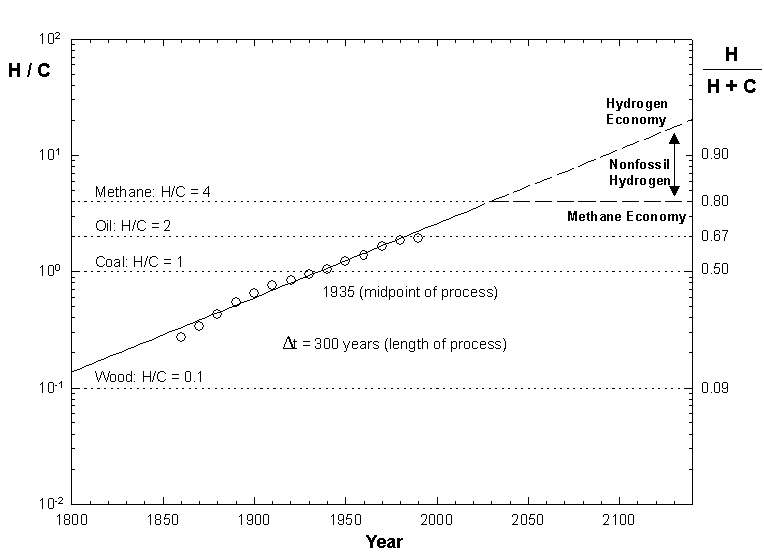
World primary energy sources have declined in carbon intensity since 1860. The evolution is seen in the ratio of hydrogen (H) to carbon (C) in the fuel mix, graphed on a logarithmic scale and analyzed as a logistic growth process. The data and logistic curve are plotted using a transform that renders the S-shaped logistic linear.
Source of figure: J. Ausubel, “Can Technology Spare the Earth,” American Scientist 84:166-178 (March-April 1996).