Citation: Technology in Society 22: 289–302 2000
Keywords: Forests, land use, agriculture, fisheries, oceans
Areas of Research: Technology & Human Environment
This essay was first prepared as a keynote address entitled “Robust Earth”, presented at the Open Science Conference of the Global Change Program (GCTE & LUCC) on 16 March 1998 in Barcelona, Spain. The marine section of the paper was added after the Barcelona meeting. The final version of the paper appears in the journal Technology In Society, Vol. 22:289-301, 2000.
In the middle of the 20th century, humans began to reverse the pattern they followed for millennia of extending further into nature to meet needs for food and materials. Recognizing this Great Reversal, I explore the areas in human use for cities, logging, and farming and search the centuries for principles and trends to forecast land use in the latter part of the 21st century when global population may number 10 billion. Offsetting the sprawl of cities, rising yields in farms and forests and changing tastes can release large amounts of land. For example, with growing population and cities, the USA in the next century could still newly spare for nature an area twice the size of Spain. Shifting from hunting to farming fish can similarly spare nature. Globally, wise and intelligent humanity can extend the Great Reversal into a Great Restoration of nature on land and in the sea.
Introduction
The American writer Gertrude Stein remarked around 1930 that the United States was already the oldest country in the world, because it had been in the 20th century longer than any other. Studying changes in the American landscape, I have become convinced that a Great Reversal is underway. For centuries Americans extended into the landscape as they became more numerous and sought more food, fuel, fiber, and other materials. In about 1950 Americans began to contract. In this essay I explore the global chance for a Great Reversal. This Great Reversal might liberate the environment from an important fraction of the disruption that humans cause.[1]
I simplify my task by focusing on area, hectares or square kilometers. I believe an areal measure of land actively used for cities, logging, and farming is the best single measure for environmental impact. Generally speaking, the smaller the total area in active human use, the more environmentally friendly will be the landscape.
To show why and how we can spare land, I will search the past century for principles and trends influencing building, logging, and farming and contemplate about 70 years into the future, when people may number 10 billion. Then I extend the logic to the oceans. Because the USA may presage the future, many of my examples are American.
Cities
The built environment, “cities” for short, includes land not only for roads, shopping centers, and dwellings, but also for lawns, town gardens, and parks. In the USA the covered land per capita ranges from about 2,000 m2 in states where travel is fast, such as Nebraska, to about 600m2 in slower, more urban New York.[2] The 30 million Californians, who epitomize sprawl, in fact average 628m2 of developed land each, about the same as New Yorkers.
The transport system and the number of people basically determine covered land. Greater wealth enables people to buy higher speed, and when transit quickens, cities spread. Both average wealth and numbers will grow, so cities will take more land.
What are the areas of land that may be built upon? The USA is a country with a fast growing population, and expects about another 100 million people over the next 70 years. At 600m2 each, the USA increase would consume 6 million hectares, about the land area of Belgium plus the Netherlands or five Connecticuts. Globally, if everyone new builds at the present California rate, the 4 billion added to today’s 6 billion people would cover about 240 million hectares, midway in size between Mexico and Argentina.
By enduring crowding, urbanites spare land for nature. In fact, migration from the country to the city formed the long prologue to the Great Reversal. Towering urbanites could spare more land. Still, cities will take from nature. Can changes in logging and farming offset the urban sprawl?
Forests
To shed light on changes of forested area, I ask first does multiplying the number of people or wealth equally multiply the use of the products taken from the forest? The answer to this question comes by dissecting historic growth in demand. This growth is the product of an identity: population multiplied times GDP per person multiplied times the timber product per GDP.
First consider the USA consumption of the four timber products: lumber, plywood and veneer, pulp products, and fuel.[3] Between 1900 and 2000 the national use of timber products grew about 70%. Large features of the century include the big growth of pulp—that is, paper and paperboard—and the small growth of lumber. Fuel wood use nearly disappeared and then re-emerged, mostly to power pulp and paper mills. Plywood consumption emerged but remained small.
The preeminent feature is that the consumption of timber products rose far less than the rises in population and wealth might suggest. At the end of the century, Americans numbered more than three and a half times as many as at the beginning, and an American’s average share of GDP had grown nearly five fold. Had timber consumption risen in constant proportion, Americans would have consumed about 16 times as much timber each year in the 1990s as in 1900, rather than the 1.7 times they actually consumed.
The explanation for the difference lies largely in the third term in the identity mentioned above, the product consumed per unit of GDP, for example, pulp/GDP. If this term, which I will call “intensity of use”, is constant, then consumption will rise in unchanging proportion to the combined rise of population and wealth.
Practically, what changes timber product per GDP? In the case of lumber, its replacement during the century by steel and concrete in applications from furniture and barrels to cross ties and lath lowered the intensity of use. Living in the stock of existing houses and prolonging the life of timber products by protecting them from decay and fire lower it. In the case of pulp, more widespread literacy and the shift to a service economy raised the intensity of use in the early 20th century. Thicker paper replaced thinner paper, and newspapers replaced oral gossip. More recently, thinner paper has again replaced thicker paper, and television has replaced newspapers, lowering the intensity of pulp per GDP. More generally, the onset of dematerialization, as telephones and magnetic files replace letters and manuscripts, is lowering it. Because both writing and packaging consume much pulp, both are opportunities for further improvements in intensity of use.
Overall, history shows the extent of forests in the USA changed little in the 20th century (Figure 1). Meanwhile, reversing hundreds of years of depletion, the volume of wood on American timberland has actually risen, by 36% since 1950. The main reason the forest has grown rather than shrunk is that on average a contemporary American annually consumes only half the timber for all uses as a counterpart in 1900. Meanwhile millers learned to get more product from the same tree, and foresters grew more wood per hectare. Already many areas initially cleared have regenerated, as evidenced by today’s large wooded areas in New England and the upper Great Lakes states. What is the woodland prospect?
Great opportunities seem open for forestry to raise yields and so further decouple demand for timber from demand for land. On a hectare of USA timberland, annual growth currently averages about 3 m3 of wood. Rates 10 to 20 times faster have been reported for trees as diverse as alder, poplar, eucalyptus, hemlock, and loblolly pine. Strategies as simple as ridges to improve drainage in wet soils speed growth. Sylvaculture could be at the beginning of a march of rising yields as agriculture was about 1940.
In the USA the plausible anticipation of a falling intensity of use for forest products should more than eliminate the effects of growing population and affluence, leading to an average annual decline of perhaps 0.5% in the amount of timber harvested for products. A conservative 1.0% annual improvement in forest growth would compound the benefits of steady or falling demand and could shrink the area affected by logging 1.5% annually. Compounded, the 1.5% would shrink the extent of logging by half in 50 years. If only one half of this amount occurs by leaving areas now cut uncut, the area spared is 50 million hectares, 8 times the area USA cities will cover, and about the combined area of the States of Washington, Oregon, and Maine, or the size of Spain. Compounding 20 more years would spare 20 million more hectares.
If we accept Gertrude Stein’s view of the American experience, forest regrowth appears part of modernity. In fact, studies of forest biomass for the decade of the 1990s in the boreal and temperate region in more than 50 countries show the forests expanding in every one.[4] Globally, rising productivity of well-managed forests should comfortably allow 20% or less of today’s forest area of about 3 billion hectares to supply most world commercial wood demand sustainably in the middle of the 21st century.[5] Wise and intelligent logging advances the Great Restoration.Farms
Farms
Now consider farms. Although farmers cannot recreate virgin land, they can allow wide returns of land to nature by the steady movement toward landless agriculture. For millennia land equated in stable proportion with food, so more mouths meant more hectares farmed, and land cropped per person expanded when each mouth sought a more ample diet. Then, in a global Great Reversal, fifty years ago farmers stopped plowing up more nature per mouth (Figure 2).
Yields per hectare measure the productivity of land and the potential for the Great Restoration. During the past half century, ratios of crops to land for the world’s major grains-corn, rice, soybean, and wheat-climbed fast and globally. Per hectare world grain yields rose almost 2 percent annually between 1960 and 1997. Between 1971-1995 the yields for an index of all crops rose annually about 1.7 percent in the USA and 2.1 in Mexico while speeding upward 2.8 percent in India and 3.4 in both Indonesia and China.
As fast as farmers advance, their horizon keeps opening. Take the case of corn. Since 1960, while rising in tandem, the average world farmer grew about half the corn per hectare of the average Iowa farmer, and the average Iowa farmer grew about half the corn of his most productive neighbor. The 1990s began in Iowa with an apparent corn ceiling of about 15 tons per hectare (t/ha). But master farmers thrust through this and subsequent ceilings six times in the decade. In 1999 the Iowa master corn grower, Mr. Francis Childs, broke the state record with 24.7 t/ha.[6] In fact USA farmers achieved many corn yield records in 1999, though faced with huge weather obstacles including late planting due to spring rains in some areas and severe drought later in the growing season in others.
Mr. Childs fertilized abundantly, inspected the growing crop more than twenty times, and controlled pests. He grew his crop without irrigation. He did grow three times as many plants on each hectare as his grandfather would have grown. Mr. Childs and his fellow Iowans do not monopolize high yields. Winners in carefully scrutinized national contests have regularly exceeded 20 t/ha since 1996 in locales as diverse as arid Tonopah, Arizona and changeable Sterling, Nebraska.
The diffusion of current best practice will occupy farmers for many decades. Because improving 2 percent per year means a doubling of performance in about 40 years, on the same area the average world farmer now grows about the same amount of corn as the average Iowa farmer grew in 1960, while the average Iowa farmer now grows about the same amount his most productive neighbor achieved in 1960.
The productivity gains have stabilized global cropland since mid-century, as shrinkage in nations as diverse as the USA, Italy, and Colombia have offset expansion in Brazil, Tanzania, and elsewhere. In essence, a cluster of innovations including tractors, seeds, chemicals, and irrigation, joined through timely information flows and better organized markets, raised the yields to feed billions more without clearing new fields.
Will high-yield agriculture tarnish the land? Farmers do many things on each area of land that they crop. In general, higher yields require little more clearing, tilling, and cultivating than lower yields. Protecting a plot of lush foliage from insects or disease requires only a little more pesticide than does sparse foliage. Keeping weeds from growing in deep shade beneath a bumper crop may require less herbicide per field than keeping them from growing in thin shade. The amount of water consumed is more or less the same per area whether the crop is abundant or sparse. Growing higher yields distills away only a little more water and leaves only a little more salt than lower yields.
Seed is planted per plot; choosing a higher yielding variety need not affect the surroundings. If the improved variety resists pests, it lessens the external effects of pesticides compared to a sprayed crop. By minimally changing the external effects of things that farmers do per area, lifting yields will thus lower effects per unit of yield.
Per plot, farmers do use more of some things, such as fertilizer, to raise the yield of their crops. For example, they have applied more nitrogen fertilizer per plot to raise yields. Americans appear to have reached or passed the point of diminishing returns for applications of nitrogen fertilizer. Since 1980 in the USA absolute nitrogen use has been level and per crop has declined. In fact, the key to lifting yields is usually the sound, complementary use of varieties, water, and fertilizer, and the declining fertilizer per USA crop production indicates better management of complementary factors. Globally, nitrogen use peaked in 1988, and the sum of world fertilizers, including phosphates and potash, remains about 10% below the peak a decade ago.
If land used for farming shrinks, water use will also tend to fall, although the fraction that is irrigated will rise. In the USA, where farmers use the largest share of water, both rising withdrawals and consumption reversed about 1970 (Figure 3). Despite gains in water use efficiency, the United States is far from most efficient practice. Water withdrawals for all users in the OECD countries range tenfold, with the USA and Canada the highest. Allowing for national differences in the major uses (irrigation, electrical cooling, industry, and public water supply), large opportunities for reductions remain.
Some blame meat for eating land. Like the demand for forest land, land used for meat is the product of an identity: population times wealth ($/person) times diet (kg meat/$) times feed conversion efficiency (kg feed/kg meat) times 1/crop yield (hectares per kg of feed). In the USA between 1967 and 1990, while MacDonald’s multiplied, US land used to make meat shrank. Population and wealth increased, but diet favored meat less, the feed needed to make meat declined, and the hectares needed to grow the feed lessened as yields rose. Net, about 2% less US land each year made meat.
Let me introduce one caution here: variability. The largest deviation of the Iowa average corn yield doubled from about 20% in the 1960s and 1970s to about 40% in the 1980s and 1990s. The largest deviation of a winning Master yield was only 7% during the earlier decades but was 27% during the later ones. Reducing the growing variability and lifting the mean challenge us to make the Restoration great.
Globally, the future for both lifting means and reducing variability lies with precision agriculture. This approach to farming relies on technology and information to help the grower use precise amounts of inputs—fertilizer, pesticides, seed, water—exactly where they are needed. Precision agriculture includes grid soil sampling, field mapping, variable rate application, and yield monitoring—tied to global positioning systems. It helps the grower lower costs and improve yields in an environmentally responsible way. At a soybean seminar in Dayton, Ohio, covered by the Associated Press on 10 February 1997, American farmers reported using one-third less lime after putting fields on square-foot satellite grids detailing which areas would benefit from fertilizer.
Technology revolutionized agriculture twice in the 20th century. The tractor and other machines caused the first. Nitrogen and other chemicals were responsible for the second. The third agricultural revolution is coming from information. What do the past and future agricultural revolutions mean for land?
US farmers, by raising grain yields, have spared about 150 million hectares since 1940 from what otherwise would have been needed: an area 3 times the size of Spain. Alternately, compare a US city of 500,000 people in 2000 and the same city of 500,000 people with the 2000 diet and the yields of 1920. Farming as Americans did 80 years earlier while eating as we do now would require 4 times as much land, about 450,000 hectares instead of 110,000. Looking to a US 70 years hence with 100 million more people and the 2000 diet, farmers will spare 4 times the area of Iowa or more than one Spain if they lift yields only 1%/yr.
What is the global outlook for restoration? If the world farmer reaches the average yield of today’s US corn grower during the next 70 years, ten billion people eating as people now on average do will need only half of today’s cropland. The land spared exceeds Amazonia. This will happen if farmers sustain the yearly 2% worldwide yield growth of grains achieved since 1960, in other words if social learning continues as usual. If the rate falls by one half, an area the size of India, globally, can still revert from agriculture to woodland or other uses. If the ten billion in 2070 prefer a meaty diet of 6,000 primary calories/day for food and fuel (twice today’s average primary calories), they roughly halve the land spared. A cautious global scenario of sustained yield growth and more calories still offers more than 10% of present world farmland, more than 10 Iowas or 3 Spains, for the Great Restoration.[7]
Seas
I have spoken about logging and farming the land well enough to spare habitat for nature. What about farming fish to spare fishes? Fishes here refer to cod but also other marine species from abalone to whales.
One compelling estimate of the consequences of fishing rather than farming the ocean: fish biomass in intensively exploited fisheries appears to be about 1/10th the level of the fish in those seas a few decades or hundred years ago. Diverse observations support this estimate. For example, the diaries of early European settlers describe marvelous fish sizes and abundance off New England in the 1600s. From Scotland to Japan, commercial records document enormous catches with simple equipment during many centuries. Even now, when fishers discover and begin fishing new places, they record easy and abundant catches, for example, of orange roughy on Pacific sea mounts. Also traditional scientific surveys of fish stocks indicate fewer and fewer spawning fish (mothers) compared to recruits, (their offspring). The ratio of spawners to recruits has fallen to 20% and even to 5% of its level when surveys began. Reasons abound to spare the sea as well as the land.
People know how to spare land’s animals. Many thousands of years ago our ancestors sharpened sticks and began hunting. They probably extinguished a few species, such as woolly mammoths, and had they kept on hunting, they might have extinguished many more. Then, ten thousand years ago our ancestors began sparing land animals by domesticating cows, pigs, goats, and sheep. By herding rather than hunting animals, humans began sparing wild animals, that is, nature. Today an average American annually eats 53 kgs of pork, beef, and lamb without hunting any of nature’s animals.[8] Americans also eat 27 kgs of poultry without endangering songbirds and consume 14 kgs of eggs without robbing ducks’ nests. Americans drink 266 kgs of milk in glasses or eat the equivalent cheese and ice cream without depriving calves of their mother’s milk.
In addition to the hundreds of kgs of meat and milk from the farmers on land, an American also eats meat from the fishers of the sea, but relatively little, only about 7 kgs in a year. Much of that 7 kgs, however, is taken from the wild schools of the sea, and that fraction of total diet, though small, depletes the oceans.
What does the world eat? In a year it now eats some 74 million tons of pigs; 50 of beef; and 12 of buffalo, goats, and sheep. It eats 186 million tons of poultry and 38 of eggs. Adding about 450 million tons of milk pushes the total over 800 million tons. How does world consumption of fish that depletes the oceans compare to the 800? About 80 million tons of fish are taken wild from the sea and 20 from fish farms and ranches. Although the world eats relatively more fish than Americans, the world consumption of 80 million tons of fish from the sea that depletes the oceans is still small compared to the consumption of over 800 from domesticated animals, a consumption that does not kill wild mammals or birds.
The ancient sparing of land animals by farming shows us how to spare the fish in the sea. We need to raise the share we farm and lower the share we catch.
Fish farming does not require invention. It has been around for a long time. The Chinese have been doing very nicely raising herbivores, such as carp, for centuries.
Following the Chinese example, one feeds crops grown on land by farmers to herbivorous fish in ponds. Much aquaculture of catfish near the Gulf Coast of the US and of carp and tilapia in Southeast Asia and the Philippines takes this form. The fish grown in the ponds spare fish from the ocean. Like poultry, fish efficiently convert protein in feed to protein in meat. And because the fish do not have to stand, they convert calories in feed into meat even more efficiently than poultry.[9] All the improvements such as breeding and disease control that have made poultry production more efficient can be and have been applied to aquaculture, improving the conversion of feed to meat and sparing wild fish.
Ponds are not the only arenas of aquaculture. Another form might be called fish ranching. An analogy of fish ranching might be grazing pigs. Running wild, about 10 hogs can share a hectare. Running wild, today’s world population of one billion hogs alone would require about one hundred million hectares, more than 1/5 the land of the USA. Running wild, growing herds denude landscapes. To decouple animal agriculture from damaging the land, farmers instead grow high yields of crops, such as corn and soybeans, to feed the animals.
In some fish ranching, notably most of today’s ranching of salmon, the salmon effectively graze the oceans, as the razorback hogs of a primitive farmer would graze the oak woods. Such aquaculture consists of catching wild “junk” fish or their oil to feed to our herds, such as salmon in pens. We change the form of the fish, adding economic value, but do not address the fundamental question of the tons of stocks. A shift from this ocean ranching and grazing to true farming of parts of the ocean can spare others from the present, on-going depletion.
I have already described fish farming in ponds. With due care for effluents, pathogens, and other concerns, this model can multiply many times in tonnage. In fact, with neatly closed systems on land the model is identical to much clean manufacturing except the machines are biological. Eventually we might grow fish in closed silos at high density, feeding them proteins made by micro-organisms grown on hydrogen, nitrogen, and carbon. The fish could be sturgeon filled with caviar.
The riskier and fascinating alternative, ocean farming, would actually lift life in the oceans. The oceans vary vastly in their present productivity. In many parts of the ocean crystal clear water enable a person to see 50 meters down. These are deserts. In a few garden areas, where one can see only a meter or so, life abounds. Water rich in iron, phosphorus, trace metals, silica, and nitrate makes these gardens dense with plants and animals. The “IronEx”periments of the 1990s demonstrated the extraordinary leverage of iron to make the oceans bloom.[10]
The meat productivity depends on two factors: the supply of food for fish (or shrimp, squid, or other eaters) in the garden and how efficiently the fish convert the food into meat. First consider the yield of fish food. Oceanographers estimate that as much as 60% of ocean life grows in 2% of the ocean surface. Production on average would then be more than 70 times as great in the 2% garden areas as in the 98% desert. In principle, fertilizing a nutrient-poor tropical ocean desert to the condition of, say, the Peruvian upwelling, could increase plant or phytoplankton yield more than 70 times to feed more fish.
The second factor setting productivity is the efficiency of turning phytoplankton into meat. The efficiency with which a kilogram of fish or other popular seafood emerges from a kilogram of phytoplankton depends both on the number of intermediaries or trophic levels in the system and on the conversion efficiency at each level. The range spans from a low of about 2% in the ocean deserts to 25% or more in the Peruvian upwelling and temperate shelf gardens, such as Georges Bank.[11] The difference between one hundred kilos of phytoplankton becoming 2 kgs of fish in the desert and 25 kgs in the garden is more than dozen-fold.
In the end to consider the potential of ocean farming we must multiply the potential changes in supply of food for fish by the potential changes in efficiency. If the garden can produce 70 times as much food for fish per hectare and can turn it into fish a dozen times more efficiently, an oceanic garden is potentially hundreds of times as productive as the oceanic desert. That is, adding the right nutrients in the right places might lift fish yields by a factor of hundreds. This is the way higher yields of crops per hectare and more productive farm animals have shrunken the area farmers must till to feed the world. And so spared land for nature.
Challenges abound because the ocean moves and mixes, both vertically and horizontally. Nevertheless, technically and economically promising proposals exist for farming on a large scale in the open ocean with fertilization in deep water. (The deep ocean currents can process the resulting rain of organic materials without becoming anoxic.) One kg of buoyant fertilizer, mainly iron with some phosphate, could produce a few thousand tons of biomass.[12]
Stimulating the growth of marine plants is the crucial first step to greater productivity. Zooplankton then graze on phytoplankton and the food chain continues until the sea teems with diverse life. Fertilizing 250,000 sq km of barren tropical ocean, the size of Colorado or about 3 times the Gulf of Maine, in principle might produce a catch matching today’s fish market of 100 million tons. Colorado is less than 1/10th of 1% of the world ocean. Along with its iron supplement, such an ocean farm would annually require about 4 million tons of nitrogen fertilizer, 1/20th of the synthetic fertilizers used by all land farms.
Still another proposition would turn a problem into an opportunity. Some scientists are decrying the leaking of plant nutrients from cities and farms into estuaries and gulfs. They worry that the decay of organisms encouraged by these nutrients will consume the oxygen in the water and smother fish. In these places where nutrients are considered a problem, why not turn the nutrients into an opportunity by learning how to grow and harvest fish in those waters to relieve what we now call pollution and lessen the demand to fish elsewhere?
The point is that the high levels of harvest of wild fishes and destruction of marine habitat to capture them need not continue. The 20% of seafood already raised by aquaculture signals the potential for Reversal. Following the example of farmers who spare land and wildlife by raising yields on land, we can concentrate our fishing in highly productive, closed systems on land and in a few highly productive ocean farms. Ecological engineers can tailor closed systems to grow the particular, tasty, high-priced species. Innovative ocean farmers can make fish cakes in bulk. Humanity can act to restore the seas, and thus also preserve traditional fishing where communities value it. With smart aquaculture, we can multiply life in the oceans while feeding humanity and restoring nature.
Conclusion
The logic for Reversal and Restoration is obvious and deep. Intelligent humanity made revolutions in productivity sweep all industries in the 20th century. We now stamp out cars like tin ducks and microchips too. Unnoticed by many, revolutions in productivity also penetrated forestry and farming. Combined with more efficient production chains and changes in consumer taste, rising yields began to allow us to meet demand for food, fiber, and fuel while using less land: the Great Reversal. The enlarging forests and abandoned farms in the US and in many other nations show it.
Because cities will take a few hundred million hectares more land for the 10 billion people of 2070, we need the Reversal to spread to more nations and for it to extend into a Great Restoration. In the US, foresters may offer 70 million hectares for nature and farmers that much or more. The net effect should be to allow a restoration of nature on land in the US exceeding the size of 100 Yellowstone National Parks or twice the area of Spain. Regional and national case studies could build a global picture. Reflecting the diffusion of productivity through industries around the world, the Great Reversal will surely happen at different times in different places and with different potential. Setting goals, such as a 300 million hectare or 10% expansion of the world’s forest area by 2070, may help.
Accomplishing the Great Restoration is the work of the 21st century for foresters, farmers, scientists, engineers, and all the other participants in the wood and food businesses. While avoiding the dangers of intensive cultivation, wise humanity can lift average yields toward the present limits and lift the limits even more. By sparing cropland, we can also spare water and nitrogen.
In the seas, the Reversal still lies ahead, but we can glimpse it. Fishers and all those who depend on the preservation of marine life must hasten its arrival and solve the many problems on the way to Restoration.
Consumers, of course, can also do their share. Changing tastes can lessen our demands on nature. For those who choose it, a vegetarian diet roughly halves land used for food. Drinking diet cola rather than apple juice, we need no land at all.
However tastes may evolve, high yields are the best friend of habitat. Recalling Gertrude Stein’s remark, the decoupling of the economy from acreage, well on its way for fifty years in the USA, creates nature’s chance to restore land and sea.
Acknowledgements: Michael Markels, Perrin Meyer, Paul Waggoner, Brian Walker, and Iddo Wernick.
Figure 1. First Picture – Reversal and restoration of USA forests.
Second Figure – U.S. Forest Land Area 1630-1997.
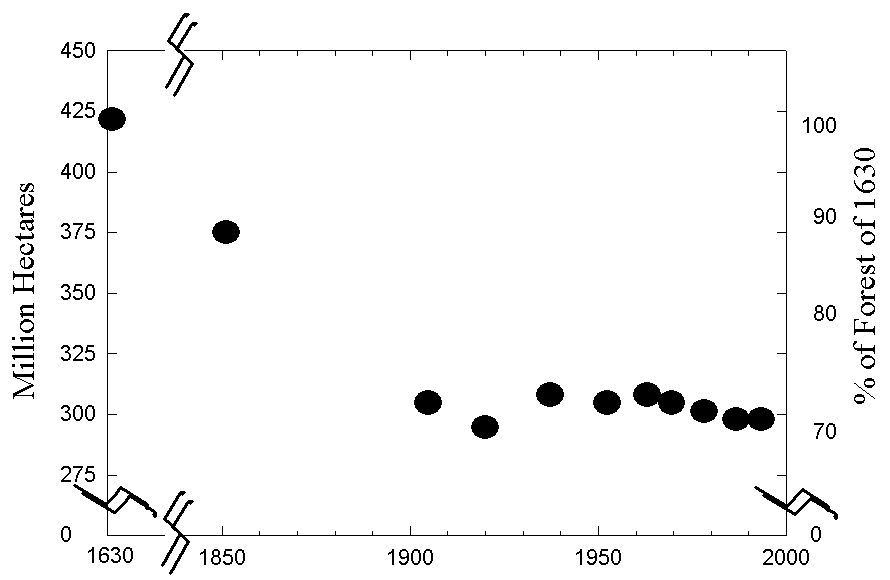
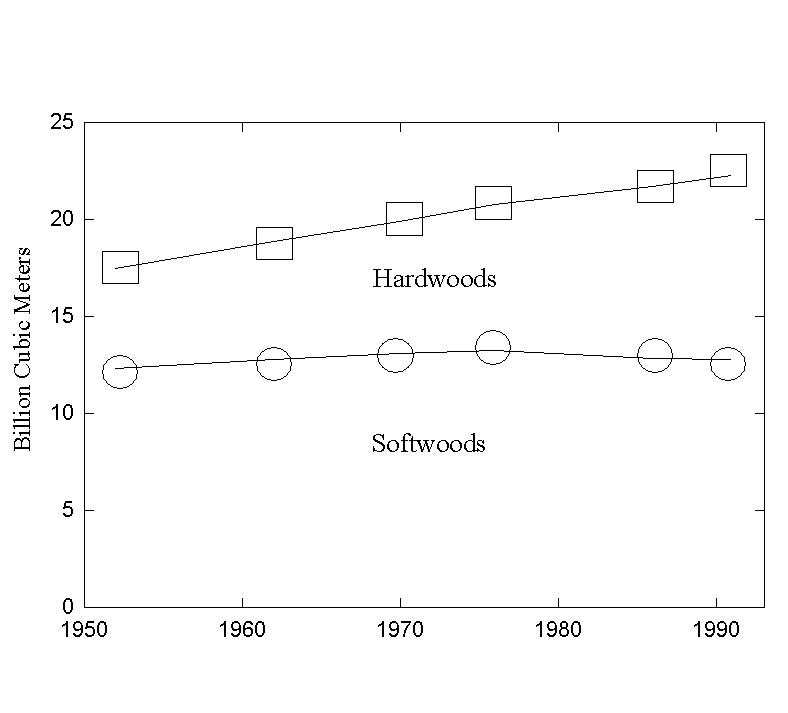
Sources of data: Sedjo (1995); Powell et al (1993). Inset: U.S. Forest Volume, Hardwoods and Softwoods, 1952-1997. Sources of data: Smith et al., 1994; Smith, 1999.
References for sources of data:
DS Powell, JL Faulkner, DR DARR, Z Zhu, and DW MacCleery. 1993. Forest Resources of the United States, 1992. USDA. Forest Service Report RM-GTR-234.
RA Sedjo. 1995. “Forests: Conflicting Signals,” in The True State of the Planet, edited
By R Bailey. New York: Free Press.
WB Smith, JL Faulkner, and DS Powell. 1994. “Forest Statistics of the United States, 1992,” USDA Forest Service Report GTR-NC-168.
Smith WB. 1997 RPA Assessment: The United States Forest Resource Current Situation. USDA Forest Service, Washington DC, 1999.
Figure 2. Reversal in area of land used to feed a person. After gradually increasing for centuries, the worldwide area of cropland per person began dropping steeply in about 1950, when yields per hectare began to climb. The square shows the area needed by the Iowa Master Corn Grower of 1999 to supply one person a year’s worth of calories. The dotted line shows how sustaining the lifting of average yields 2 percent per year extends the reversal.
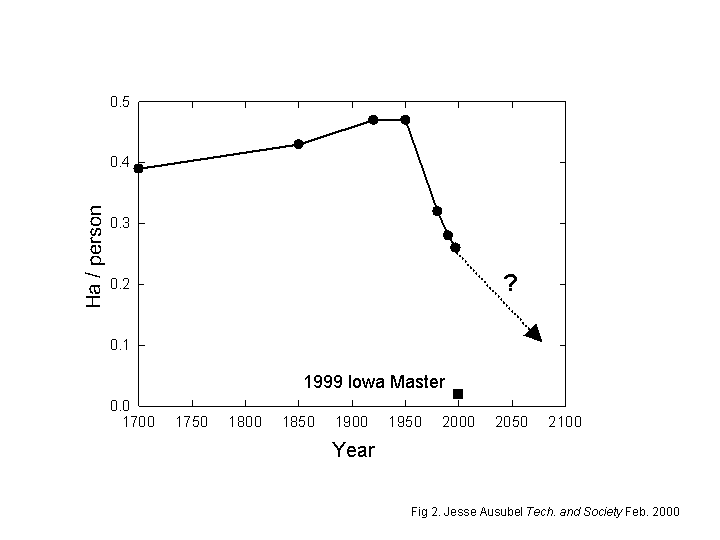
Sources of data:
Food and Agriculture Organization of the United Nations, various Yearbooks.
National Corn Growers Association, National Corngrowers Association Announces 1999 Corn Yield Contest Winners, Hot Off the Cob, St. Louis MO, 15 December 1999 ;
JF Richards. 1990. “Land Transformations,” In The Earth as Transformed by Human Action, BL Turner II et al. eds., Cambridge University: Cambridge, UK.
Figure 3. Reversal in total U.S. Water Use, Per Capita, Per Day.
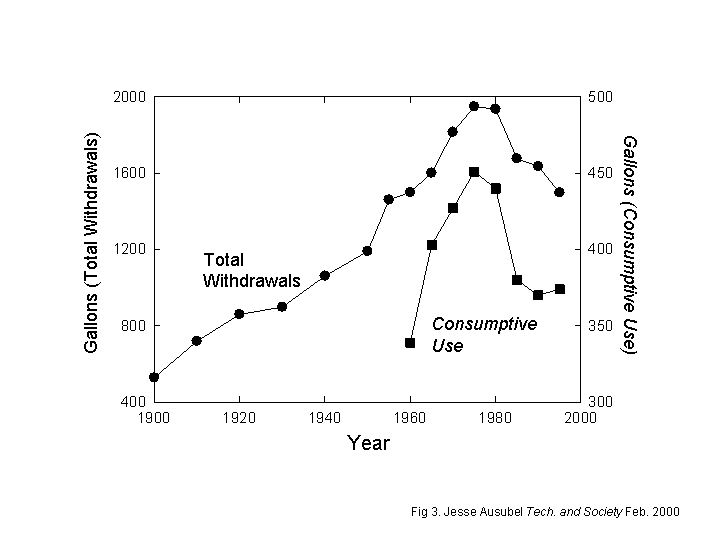
Sources of data:
U.S Bureau of the Census. 1975. Historical Statistics of the United States, Colonial Times to 1970. Washington, DC: U.S. GPO.
U.S Bureau of the Census. 1998. Statistical Abstract of the United States: 1998. (118th edition.) Washington, DC.
[1] Ausubel JH. For a discussion covering energy, materials, and other aspects along with land use see The Liberation of the Environment. Daedalus 1996;125(3):1-17.
[2] Waggoner PE, Ausubel JH, Wernick IK. Lightening the Tread of Population on the Land: American Examples. Population and Development Review 1996;22(3):531-545.
[3] Wernick IK, Waggoner PE, Ausubel JH. Searching for Leverage to Conserve Forests: The Industrial Ecology of Wood Products in the U.S. Journal of Industrial Ecology 1998;1(3):125-145.
[4] UN-ECE/FAO. Forest resources of Europe, CIS, North America, Australia, Japan and New Zealand (industrialized temperate/boreal countries), contribution to the Global Forest Resources Assessment 2000. United Nations, New York, in press.
[5] Sedjo RA, Botkin D. Using Forest Plantations to Spare Natural Forests. Environment 1997;39(10): 14-20 and 30.
[6] National Corn Growers Association, National Corngrowers Association Announces 1999 Corn Yield Contest Winners, Hot Off the Cob, St. Louis MO, 15 December 1999 ; on line at https://www.ncga.com/archives/news991215.html
[7] Frink CR, Waggoner PE, Ausubel JH. Nitrogen fertilizer: Retrospect and prospect. Proceedings of the National Academy of Sciences USA 1999;96:1175-1180.
[8] US Department of Agriculture, Agriculture Statistics. Washington DC, 1990, p. 471.
[9] Lovell T. Nutrition and Feeding of Fish, Van Nostrand Reinhold, New York, 1989. p. 6: Weight gain/g food, protein gain/g protein as follows: channel catfish 0.84, 0.36; broilers 0.48, 0.33; beef 0.13, 0.15.
[10] Martin JH et al. Testing the iron hypothesis in ecosystems of the equatorial Pacific Ocean. Nature 1994; 371:123-129.
[11] Pauly D, Christensen V. Primary production required to sustain global fisheries. Nature 1995; 374:255-257
[12] Markels, Jr., M. Method of improving production of seafood. US Patent 5,433,173, July 18, 1995, Washington DC.